- lauraclark849
- Apr 3
- 20 min read
Updated: Apr 15
Peer Review Article | Open Access | Published 2nd April 2025
Recovery of Encapsulated Bacterial Spores in Styrene-Butadiene Copolymer to Validate Blow-Fill-Seal Processes
Michael Karl Jahn¹, Marcel Goverde², Samira Giger², Natalie Reinhardt¹, Bernadette Bauer¹ | EJPPS | 301 (2025) |https://doi.org/10.37521/ejpps30102 | Click to download pdf
Abstract
In this study, a detection method for Bacillus atrophaeus spores encapsulated in blow-fill-seal (BFS) containers made from extruded copolymer was developed and validated. Bacillus atrophaeus spores, known for their high thermal resistance, serve as model organisms for evaluating sterilization efficacy in polymer extrusion processes. A method for quantifying viable spores post-extrusion was established. The usually used low density polyethylene (LDPE) 3020D was replaced by comparable Styrolux® 656C styrene-butadiene copolymers (SBC) that could be dissolved at room temperature using toluene. Finally, SBC samples could be dissolved in toluene and B. atrophaeus spores were added to check the recovery rate. A standard filtration was developed – which did not reduce the bacterial spore count – using a Milliflex Plus Pump Single Head Kit with PVDF filters including rinsing with ethanol and NaCl-peptone buffer to reactivate bacterial spores.
All positive controls showed a good recovery rate while the treatment with toluene alone yielded a recovery rate of 30% to 52%. This reduction decreased when the SBC was added to the solvent toluene giving a recovery rate of 57%. The method developed thus yielded an acceptable recovery of bacterial spores of B. atrophaeus with the copolymer Styrolux® 656C and can therefore be used to enumerate bacterial spores in BFS-manufactured containers to evaluate the efficacy of the extrusion process in eliminating microorganisms, allowing determination of the corresponding killing kinetics.
Key words: Blow-Fill-Seal technology, biological indicator, Bacillus atrophaeus, styrene-butadiene copolymer, recovery rates, pharmaceutical primary packaging
1. INTRODUCTION
The use of blow-fill-seal (BFS) technology in pharmaceutical manufacturing is a well-established method for aseptic packaging that combines the formation, filling, and sealing of containers in a single, continuous process. This technology is critical in the production of sterile liquid medications or gels due to its efficiency. It also offers enhanced product safety, reduced material waste, and lower production costs, along with the benefits of in-line sterilization and automation. However, it presents unique challenges in ensuring that stringent sterilization standards are consistently met¹ ².
According to the new Annex 1, the BFS extrusion system's capabilities must be thoroughly understood and validated. The polymer material should undergo testing for bioburden and endotoxins or pyrogens (EudraLex 2022, §8.112 and §8.114)³. During equipment qualification, it is essential to identify critical process parameters, including temperature, speed, and extruder throat settings for parison thickness (EudraLex 2022, §8.115)³. Thus, BFS systems must undergo rigorous validation to confirm that microbial inactivation is achieved during the extrusion process in which the container is formed out of polymer granulates. This step is especially important because it involves exposure to high temperatures and pressures, which play a critical role in microbial decontamination within the process.
To validate decontamination within BFS processes, Bacillus atrophaeus spores are frequently used as test organisms. As per European Pharmacopoeia⁴ or United States Pharmacopeia⁵ and contemporary scientific standards, these spores are widely recognized for their dry thermal resistance⁶. The ability of B. atrophaeus spores to withstand extreme conditions allows them to serve as a robust indicator of sterilization effectiveness under the high temperature demands of BFS extrusion processes.
To date, studies validating BFS extrusion processes have been conducted using a polymer inoculated with B. atrophaeus spores. This polymer was then used to perform an aseptic process simulation (APS) on BFS equipment³ ⁷. The units produced in this manner are subsequently incubated under standard incubation conditions (normally 7 days at 20-25 °C followed by 7 days at 30-35 °C) and checked for microbial growth⁸ ⁹ ¹⁰.Using this approach, different concepts were developed to define the sterility assurance level (SAL) of 10⁻⁶ as required by the standards⁹ ¹¹ ¹².
One of the main challenges in such studies is how to recover the Bacillus spores on and especially encapsulated in the polymer. Using an APS will only detect the spores on the inner surface of the BFS containment and probably up to 3 µm within the inner polymer wall¹³.
To recover microorganisms, traditional growth-based methods such as membrane filtration or plate count can be used. These methods are well described and validated according to pharmaceutical standards. On the other hand, alternative or rapid methods (e.g. confocal laser scanning microscopy (CLSM) with fluorescence labelled spores, qPCR, fluorescence in situ hybridization (FISH) or DNA extraction) can be used. For example, FISH in combination with CLSM can be used to stain microorganisms on and to a certain degree within polymers. However, the method is time-consuming and needs specific validation if used under GMP (Good Manufacturing Practice) conditions¹³ ¹⁴. Since the pharmacopoeias use growth-based methods⁵ ¹⁵ ¹⁶ ¹⁷, it is easier to work with the traditional growth-based methods under GMP as they are considered validated and therefore only need to be verified. Therefore, the aim of the present study was to develop a method to enumerate B. atrophaeus spores encapsulated in the polymer of BFS containers.
The challenge of recovering encapsulated microorganisms is analogous to the difficulties faced in spacecraft contamination studies, where the polymers frequently used in spaceflight hardware could pose a risk of contaminating extraterrestrial environments. Baumeister et al. (2014)¹³ investigated five different polymers inoculated with Bacillus safensis spores as well as different methods, including dissolving the polymer and enumerating the spores with traditional growth-based methods but also with alternative methods such as qPCR and CSLM. For the traditional growth-based approach, they dissolved the polymer with different solvents such as acetone, propanol, and ethanol and found recovery rates from 12% up to 89%.
Various solvents for dissolving polymers have been tested by different researchers¹³ ¹⁴ ¹⁸ ¹⁹ and some of them have only a small negative effect on bacterial spores and sometimes only a negative effect of 1-log reduction on vegetative cells¹⁴ ¹⁸.
In the present study, a method was developed to enumerate encapsulated B. atrophaeus spores in polymer granulate used for the BFS extrusion processes using traditional growth-based methods described by the pharmacopeia.
2. METHODS
Test organisms and granulate inoculation
A spore suspension of Bacillus atrophaeus was prepared at an initial density of 4.4×10⁸ spores/mL in sterile ethanol (96% (v/v), EMPROVE® EXPERT Ph. Eur., JP, USP, SAFC®, VWR Code 1.00967.2500). The suspension was subsequently diluted with 96% EtOH to a level of 4.4×10² spores/mL to obtain quantifiable microbial counts within the range of approximately 10 to 100 colony-forming units (CFU) per sample and stored at 2-8 °C. Over time, spores tend to aggregate, and vortex mixing alone was insufficient to gain homogeneity. Therefore, prior to use, the suspension underwent 10 minutes ultrasonication (640 W, 35 kHz) and vortex mixing to ensure homogenous spore distribution before inoculation onto BFS granulate.
B. atrophaeus are rod-shaped, gram-positive organisms. Colonies on casein soya bean digest agar (CASO) have a characteristic brown, rubiginous color with a darker coloring in the center.
Extruder process
The extrusion process for blow-fill-seal (BFS) involves melting plastic such as polyethylene or polypropylene. The process begins with feeding plastic granulates into the granulate hopper of the BFS machine for the extrusion of plastic containers, where they are melted and conveyed by rotating screws. The melted plastic is then extruded through a nozzle to form a parison, which is shaped into a container via blow molding in a sterile environment, minimizing contamination risks² ⁸ ¹⁰.
Preliminary tests
LDPE used for BFS processes dissolves well with toluene or benzene at approximately 80 °C, but since benzene is more dangerous to handle and is carcinogenic, toluene is the preferred solvent¹⁸. Therefore, in the present study, primarily toluene was used. Furthermore, it was shown that microorganisms and especially bacterial spores, can be kept well in toluene and have a low mortality²⁰ ²¹.
A series of preliminary tests were performed to find an appropriate polymer that could be used in the BFS process, but which could also be used to recover the inoculated bacterial spores. For this, different polymers were tested.
Test with low density polyethylene 3020D
In primary experiments, low density polyethylene 3020D (LDPE 3020D, Lyondell Basell Industries Wesseling, Article-No.:10169A12) was dissolved in toluene at temperatures above 80 °C. Below 80 °C, the dissolved LDPE 3020D rapidly precipitates, leading to two primary challenges:
Throughout the filtration process to recover the bacterial spores, both the LDPE solution and filtration apparatus must remain above 80 °C to avoid precipitation. To establish such a hot-filtration setup capable of maintaining a stable temperature throughout filtration under standardized conditions is very challenging.
The elevated temperature required for dissolution and subsequent hot filtration significantly reduces the viability of spores by 83-87%.
Consequently, this technique could not be reliably or reproducibly conducted under laboratory conditions and the lethality for the spores was high. In conclusion, the use of LDPE 3020D was discarded for the present study approach.
Tests with different types of styrene-butadiene copolymers
As an alternative to the LDPE, the dissolution of various styrene-butadiene copolymers (SBC, Styrolux®, INEOS Styrolution Group GmbH, Frankfurt am Main) in toluene at room temperature was investigated. Different types of SBC were evaluated for their color, solubility and filterability. The filtration of white-colored SBC led to immediate filter blocking, making this process infeasible. The filtration of transparent SBC worked relatively well, although filter blocking did occur over time, depending on the SBC concentration and volume of solution (Table 1). Following multiple trials, SBC type 656C was identified as the most suitable in terms of its color, solubility and filterability using the Milliflex Plus filtration system with polyvinylidene fluoride (PVDF) membranes and polypropylene funnels, both toluene-resistant, gamma-sterilized and provided as ready-to-use units. As an alternative, 3G46 could also be used but this SBC has a higher butadiene concentration than 656C, and therefore the former should be easier to filter.
Table 1: Overview of the different types of SBCs and their behavior.
SBC | Color | Dissolution | Filtration |
656C | Transparent | Easily achievable | Well filtrable |
684D | Transparent | Easily achievable | Slowly filtrable |
3G46 | Transparent | Easily achievable | Well filtrable |
486N | White | Achievable, opaque solution | Not filtrable |
454N | White | Achievable, opaque solution | Not filtrable |
495N | White | Achievable, opaque solution | Not filtrable |
Concentration of SBC
To find the best concentration of dissolved SBC 656C in toluene, two different concentrations were tested. While the 1.0 g/100 mL showed low filterability, the 0.5 g/100 mL showed good filterability with a maximum of 7 minutes filtration time.
Solvent for homogenate
Since SBC 656C needed to be dissolved in toluene for 20 minutes and this decreased the viability of the bacterial spores by approximately 60%, another diluent, the solvent D-limonene, was evaluated ((R)-(+)-Limonen p.a., Material-No. 81840705500, Merck KGaA, Darmstadt). However, D-limonene gave lower bacterial recovery, needed an extended incubation time before colonies were visible on solid agar, and provided slower and poorer dissolution of SBC 656C.
Therefore, solvent toluene was seen as the best option for dissolving SBC 656C.
Method development
All dissolution and filtration experiments were performed under a fume hood after careful disinfection.
Standard filtration
The following standard filtration was developed and then used:
The Milliflex Plus Pump Single Head Kit (DEM-MXPPLUS01) using a PVDF filter was pre-treated with 50 mL of toluene (Merck KGaA, VWR Code 1.08325.1000) to make the filter surface hydrophobic.
The sample solution was then filtered.
The filter membrane was dried on the pump for 2 minutes to eliminate residual toluene and any remaining sample material.
The filter was then rinsed with 50 mL of 96% ethanol to remove residual toluene. This ethanol rinse also re-hydrophilizes the membrane and facilitates subsequent rinsing steps with NaCl peptone buffer (buffered sodium chloride peptone broth, pH 7.0, according to Ph. Eur. 2.6.13²², VWR Code 84605.0500).
A final rinse with 500 mL of NaCl peptone buffer was applied to aid the recovery of bacterial spores, which may have experienced growth inhibition due to prior exposure to toluene.
Upon completion of filtration and rinsing, the vacuum pump was deactivated, initiating an automatic "dry out" cycle to thoroughly dry the filter membrane.
The filters were then transferred to Milliflex Pre-filled Media Cassettes (casein soya bean digest agar, Merck KGaA, Catalogue Number: MXSMCTS48) and incubated for 5 days at 30-35 °C.
Negative controls with toluene
To detect potential sources of contamination or issues in executing the defined validation protocol, a negative control was performed without spore addition or polymer.
For this purpose, 100 mL of toluene, free from spores, was placed in a sterile 250 mL Schott bottle and stirred on a magnetic stirrer for 20 minutes. This 20-minute exposure simulated the process of SBC dissolution in toluene.
Following the exposure period, the sample was filtered according to the standard filtration method and the filter transferred to Milliflex Pre-filled Media Cassettes and incubated as described above.
Negative control with SBC
A negative control was performed without spore addition but with the inclusion of SBC granulate. A total of 100 mL of toluene, without spore addition, was added to a sterile 250 mL Schott bottle along with 0.5 g of SBC 656C granulate. The mixture was stirred on a magnetic stirrer for 20 minutes. Following this, the sample was filtered according to the standard filtration method and the filter transferred to Milliflex Pre-filled Media Cassettes and incubated as described above.
Inoculum controls
The validation process included recovery assessments of CFUs in comparison to an inoculum control, adhering to the acceptance criterion outlined in Ph. Eur. chapter 2.6.1216. According to this monograph, an acceptable recovery range is between 50% and 200%. If recovery values fall outside this range, a correction factor can be applied as per ISO 11737-1, Annex C (2018)²³.
A spore suspension containing approximately 10² CFU/mL was prepared. For each assay, 200 μL of this dilution was tested using the surface spread method with a Drigalski spatula. To control variability and enable averaging, five replicate CASO agar plates (Merck KGaA, Article Code: 146004) were prepared, each receiving 200 μL of the vortexed spore suspension (vortexed for 1 minute prior to plating). The plates were incubated as described above.
Positive control NaCl peptone buffer
This experiment aimed to evaluate the intrinsic effect of filtration in comparison to the Drigalski control (inoculum control). The setup included 100 mL of NaCl peptone buffer with the addition of spores. Filtration was carried out without the toluene pre-wetting step, deviating from the developed standard filtration protocol. CFU recovery was expected to align with inoculum control results.
For each setup, 100 mL of NaCl peptone buffer was transferred to a sterile container, followed by the addition of 200 μL of a spore suspension (10² CFU/mL). The mixture was homogenized using a vortex mixer. Before filtration, the funnel was pre-wetted with 100 mL of NaCl peptone buffer and after adding the sample it was rinsed with an additional 300 mL of NaCl peptone buffer. A total of three replicates were performed. The filters were transferred to Milliflex Pre-filled Media Cassettes and incubated as described above. The recovery rate indicated in Table 2 was calculated using the following formula:

Positive control toluene wetting
This positive control was designed to assess the influence of the toluene wetting step on the membrane during the subsequent standard filtration process outlined above. As the base medium, NaCl peptone buffer was used. Each replicate was supplemented with 200 μL of spore suspension and subjected to the developed standard filtration method. Three replicates were performed, each involving 100 mL of NaCl peptone buffer with 200 μL of spore dilution (10² CFU/mL). The mixtures were homogenized prior to filtration following the standard protocol. The filters were transferred to Milliflex Pre-filled Media Cassettes and were incubated as described above. The recovery rate indicated in Table 2 was calculated using the following formula:

Positive control toluene dissolution (toluene with spores and standard filtration)
To assess the effect of SBC dissolution time in toluene, 100 mL of toluene was combined with 200 μL of spore dilution, stirred for 20 minutes, and processed using the standard filtration protocol.
The experiments were performed in triplicate. 100 mL of toluene was placed in each of three clean 250 mL Schott bottles, followed by 200 μL of a spore suspension at 10² CFU/mL. The samples were stirred on a magnetic stirrer for 20 minutes, then filtered using the standard filtration protocol with a Milliflex pump. The filters were transferred to Milliflex Pre-filled Media Cassettes and incubated as described above. The recovery rate indicated in Table 2 was calculated using the following formula:

SBC dissolution process
The experiments were conducted in triplicate. For each replicate, 100 mL of toluene was added to a 250 mL Schott bottle, equipped with a magnetic stir bar, followed by the addition of 200 µL of a spore suspension containing 10² CFU/mL. Subsequently, 0.5 g of SBC 656C, cut into pieces of approximately 0.5 cm in edge length, was introduced into the suspension. Care was taken to add the SBC particles individually to minimize clumping. The samples were stirred for 20 minutes and then filtered using the standard filtration procedure. The filters were transferred to Milliflex Pre-filled Media Cassettes and incubated as described above. The recovery rate indicated in Table 2 was calculated using the following formula:

RESULTS
Negative controls
Neither approach for the negative controls showed any microbial growth, thereby showing that neither the manipulation, filtration unit, solvents and buffers used, nor the SBC granulate had any microbial contamination.
Positive controls without SBC
Table 2 summarizes the different controls which were performed to evaluate the different effects on the B. atrophaeus spores. The inoculum controls using 200 μL direct surface spread plating gave an average of 75 CFU.
Spores in NaCl peptone buffer and filtered with NaCl peptone buffer, showed a recovery rate of 113% compared to the inoculum control. This difference to the inoculum control is minimal and is well within the acceptance criterion of 50-200% used in the present experiments.
Pretreatment of the filter with toluene did not significantly influence the recovery rate (recovery rate of 105% in Table 2). In contrast, samples exposed to toluene for 20 minutes exhibited a significant reduction in spore viability, with recovery rates dropping to 52% (Table 2), demonstrating the adverse impact of extended toluene exposure of B. atrophaeus spores. Therefore, a correction factor could be applied as described in ISO 11737-1, Annex C (2018)23:

Table 2: Calculation of recovery rates of the different positive controls without SBC 656C.
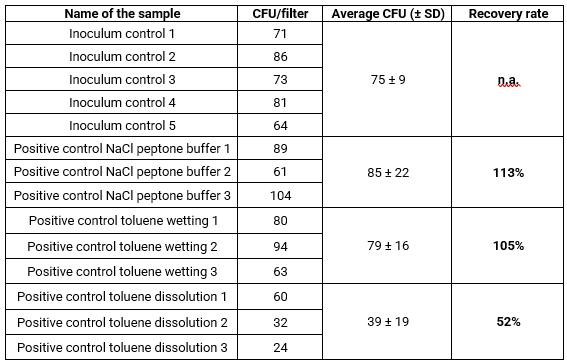
Evaluation of results from tests with SBC addition
Table 3 summarizes the data for the tests with the SBC. The inoculum controls using 200 μL direct surface spread plating gave an average of 79 CFU which is in line with the first inoculum control of 75 CFU.
As demonstrated with the control tests in Table 2, the recovery rates of CFUs from samples treated with toluene for 20 minutes revealed a significant negative effect of prolonged toluene exposure on spore viability and resulted in a recovery rate of only 30% (Table 3).
For samples in which 0.5 g of SBC 656C was dissolved in toluene over 20 minutes, the recovery rate underscored the impact of toluene on viability, resulting in a 57% recovery rate relative to the inoculum control. However, this recovery rate was significantly higher than that of spores exposed solely to toluene for 20 minutes, where 52% was recovered in the first experiments (Table 2) and 30% in the second (Table 3). The presence of dissolved SBC had no additional inhibitory effect on spore recovery compared to toluene treatment alone, in contrast, it increased the recovery rate by 26%.
Based on these results, as for the first set of experiments, a correction factor as recommended by ISO 11737-1, Annex C (2018)23 can be applied:

Table 3: Calculation of recovery rates with SBC 656C.
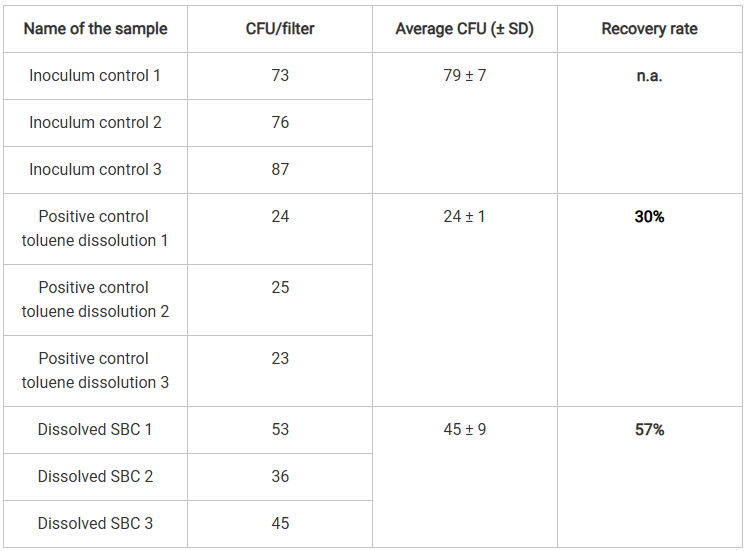
Discussion
Preliminary experiments with LDPE indicated that the material dissolves in toluene only at elevated temperatures, specifically above 80 °C. However, it was observed that such high temperatures adversely affected the viability of the bacterial spores, rendering the extraction method unreliable for viable count determination. Furthermore, the hot filtration process, while initially promising, proved challenging to standardize, with significant reproducibility issues observed across trials. An alternative to the dissolution of the polymer is to shred the material physically, for example, in a blender or with a box grater²⁴ ²⁵. However, such techniques will not be sensitive enough and with the blender there is a risk of overheating and thereby compromising the spores.
Baumeister et al. (2014)¹³ found that Bacillus safensis spores had a good recovery rate using different solvents such as acetone, propanol and ethanol at room temperature. Therefore, to increase the recovery rate, as an alternative to LDPE, different types of SBCs that dissolve in toluene at room temperature were investigated with the aim of finding a polymer that (1) can be used in the BFS process, (2) can be dissolved at room temperature, (3) is easy to dissolve (i.e. not needing too much dissolving time), (4) is well filterable (i.e. does not block the filter), and (5) delivers an acceptable recovery rate of B. atrophaeus. This was found by using the Styrolux® 656C. This material can be used for the BFS process in a similar way to LDPE and dissolves well in toluene at room temperature.
Another crucial factor was the direct impact of toluene on the viability of the spores. To mitigate the negative effects, the method was optimized with rinsing steps involving ethanol and NaCl peptone buffer. Filtration was standardized using the Milliflex Plus system with polyvinylidene fluoride (PVDF) membranes, which are resistant to toluene and equipped with sterile units. To avoid blocking the filter, the concentration of the SBC solution used was limited to 0.5 g/100 mL.
A replacement of toluene with D-limonene was tested, however, it gave lower recovery which might be due to its ability to break the potential, permeability and integrity of the cell membrane and disrupt the anabolism and catabolism in cells as shown in its fungicidal effect²⁶ ²⁷.
To reduce the negative effect of the dissolution of SBC in toluene on B. atrophaeus spore viability, a standard filtration process was established. Using the corresponding controls, it could be shown that the developed standard filtration process gives a very good bacterial spore recovery rate. Additionally, the lack of growth in the negative controls underlines that the method delivers reproducibly good values and that secondary contaminations can be excluded, although the experiments were performed under a fume hood and not under a laminar flow unit. Thus, dissolution of SBC 656C in toluene at room temperature, followed by the established standard filtration through PVDF membrane filters, facilitated the recovery of bacterial spores from the polymer matrix. However, the results of the positive control in the toluene solution demonstrated a clear negative impact of toluene on spore viability, as evidenced by a significantly lower recovery rate compared to controls where spores were suspended in NaCl peptone buffer and filtered. Bacterial spores are regarded as some of the most resilient life forms on earth, exhibiting extraordinary resistance to extreme environmental conditions. Bacillus spores, in particular, are capable of enduring heat, ultraviolet radiation, oxidative stress, desiccation, and exposure to various chemical agents such as acids, bases, phenols, alcohols, and alkylating compounds. Key factors contributing to their chemical resistance include the spore coat's impermeability to hydrophilic substances, the low water content in the spore core that keeps enzymes in an inactive state, and the protection of spore DNA by alpha-beta SASP proteins²⁸ ²⁹. However, there are limitations and if even 20-50% of spores can survive harsh conditions this represents a tremendous evolutionary advantage for this life form. In the current study, toluene had a negative effect resulting in B. atrophaeus recovery rates of 52% and 30%, respectively. Therefore, to obtain accurate and reliable spore count values, a correction factor within this method to account for the detrimental effect of toluene on spore viability can be implemented. Such a correction factor can be applied to the CFU counts of all samples that are exposed to toluene and subsequently filtered to obtain corrected microbial count values. On the other hand, with 52% the recovery is still within the requirements given in the pharmacopeia of 50-200%, while 30% is out of this range and indicates an inhibition which needs further sample treatment. However, when samples from BFS manufacturing are compared, a correction factor might not be relevant since in these cases a comparison is being made and absolute enumeration is not needed.
Interestingly, in the presence of SBC a lower reduction in spore viability was found in the system containing SBC and toluene compared to the system with pure toluene. This observation can be attributed to two potential mechanisms:
(1) Protective effect of the dissolved SBC: The dissolved SBC molecules could partially coat the spores in the solution and thus protect them from direct contact with the toluene. This would lead to less damage to the bacterial spores and thus to a higher recovery rate. Such effects are known from silicone oil and other substances that increase the resistance of bacterial spores to sterilization by increasing its D-value ³⁰ ³¹ ³².
(2) Uneven toluene distribution: It is conceivable that the toluene is not completely homogeneously distributed in the SBC solution. As a result, the spores in the solution could be exposed to toluene to different degrees, which would lead to a greater variance in spore viability and an apparently higher recovery rate compared to the approach with pure toluene.
5. CONCLUSIONS
In the present study, in a first step, the usually used LDPE polymer for BFS processes was substituted by the SBC Styrolux® 656C which dissolves well in toluene at room temperature, enabling the recovery of the bacterial spores encapsulated in the polymer. Then a standard filtration was established to enumerate the released spores. The lowest recovery was found when the spores were in contact with toluene only. As soon as the spores were dissolved in the presence of the SBC, recovery increased above 50%, which is seen as an acceptable recovery rate in pharmaceutical microbiology¹⁶ ¹⁷.
Having established this recovery method, it is now possible to use microbially contaminated granulate to evaluate the BFS extrusion system’s capability to align with regulatory requirements³ ⁷ ³³. To date, inoculated granulate was used to produce BFS containers which were filled with nutrient broth (aseptic process simulation or media fill). Using this approach, it is not possible to show a SAL of 10⁻⁶ since it would be necessary to fill and evaluate 1,000,000 units, which is not feasible.
In conclusion, using the here-presented reliable and standardized recovery method for detecting B. atrophaeus spores in BFS containers, the efficacy of the extrusion process in eliminating microorganisms can be evaluated, thereby deriving the corresponding killing kinetics for the process. Such studies were performed by the present authors and are published in a follow-up study by Geser et al. (2025)³².
Acknowledgements
Lucas Stegmeier for performing preliminary studies (published as Experiments to Develop a Method for the Recovery of Embedded Spores in Plastic. Extruder Design Space Verification for an Aseptic Filling Process with Blow-Fill-Seal Technology. Praxis Semester Report. Reutlingen University, Faculty of Applied Chemistry, 2021).
Data availability
Data will be made available on request.
Conflict of Interest
The authors declare that they have no competing interests.
The current study was performed at Holopack Verpackungstechnik GmbH, Bahnhofstraße 18, D-74429 Sulzbach-Laufen, Germany with internal funding.
References
01. Bondre S, Puttewar T, Patil RY. Review: A focus on blow-fill-seal technology. Advance Research in Pharmaceuticals and Biologicals 2013;3(IV): 494–499.
02. Baseman H. Operation and Validation of Blow Fill Seal Technology. In: Sterilization of Medical Devices [eBook], 1999. ISBN: 9780203738306.
03. EudraLex. Annex 1 – Manufacture of Sterile Medicinal Products. The Rules Governing Medicinal Products in the European Union, Volume 4, EU Guidelines to Good Manufacturing Practice, Medicinal Products for Human and Veterinary Use, 2022.
04. Ph. Eur. chapter 5.1.2. Biological indicators and related microbial preparations used in the manufacture of sterile products. Current version.
05. USP chapter <1229.5>. Biological indicators for sterilization. Current version..
06. Sella RBR, Vandenberghe LPS, Soccol CR. Bacillus atrophaeus: main characteristics and biotechnological applications – a review. Critical Reviews in Biotechnology 2015; 35(4): 533–545.
07. FDA. Guidance for Industry: Sterile Drug Products Produced by Aseptic Processing – Current Good Manufacturing Practice. U.S. Department of Health and Human Services, Food and Drug Administration, Rockville, MD, 2004.
08. Liu W, Faulhaber S, Sane SU, Lam P. Biopharmaceutical Manufacturing Using Blow–Fill–Seal Technology. BioPharm International 2011;24(7).
09. Leo F, Poisson P, Sinclair CS, Tallentire A. Evaluation of Blow/Fill/Seal extrusion through processing polymer contaminated with bacterial spores and endotoxin. PDA Journal of Pharmaceutical Science and Technology 2004;58(3):147–158.
10. Wu VL, Leo FN. Advances in Blow/Fill/Seal Technology: A Case Study in the Qualification of a Biopharmaceutical Product. In: Biotechnology and Biopharmaceutical Manufacturing, Processing, and Preservation [eBook], 1996. ISBN: 9781003076063.
11. Leo F, Poisson P, Sinclair CS, Tallentire A. Design, development and qualification of a microbiological challenge facility to assess the effectiveness of BFS aseptic processing. PDA Journal of Pharmaceutical Science and Technology 2005;59(1):33–48.
12. Bradley A, Probert SP, Sinclair CS, Tallentire A. Airborne microbial challenges of Blow/Fill/Seal equipment: a case study. PDA Journal of Parenteral Science and Technology 1991;45(4):187–192.
13. Bauermeister A, Mahnert A, Auerbach A, Böker A, Flier N, Weber C, Probst AJ, Moissl-Eichinger C, Haberer K. Quantification of Encapsulated Bioburden in Spacecraft Polymer Materials by Cultivation-Dependent and Molecular Methods. PLoS ONE 2014;9(4).
14. Mohapatra BR, La Duc MT. Evaluation of fluorescence in situ hybridization to detect encapsulated Bacillus pumilus SAFR‐032 spores released from poly(methylmethacrylate). Microbiology and Immunology 2012;56(1):40–47.
15. Ph. Eur. Chapter 5.1.3. Efficacy of antimicrobial preservation. Current version
16. Ph. Eur. chapter 2.6.12. Microbiological examination of non-sterile products: microbial enumeration tests. Current version.
17. USP Chapter <61>. Microbiological examination of nonsterile products: Microbial enumeration tests. Current version.
18. Wong SL, Ngadi N, Abdullah TAT. Study on Dissolution of Low Density Polyethylene (LDPE). Applied Mechanics and Materials 2014;695:170–173.
19. Vasin VB, Trofimov VI. The experimental study of microbial contamination of space hardware. Advances in Space Research 1995;15:273–276.
20. Johnson MJ, Todd SJ, Ball DA, Shepherd AM, Sylvestre P, Moir A. ExsY and CotY Are Required for the Correct Assembly of the Exosporium and Spore Coat of Bacillus cereus. Journal of Bacteriology 2006;188(22):7905–7913.
21. Darszon A, Escamilla E, Gómez-Puyou A, Tuena M. Transfer of spores, bacteria and yeast into toluene containing phospholipids and low amounts of water: Preservation of the bacterial respiratory chain. Biochemical and Biophysical Research Communications 1988;151(3):1074–1080.
22. Ph. Eur. chapter 2.6.13. Microbiological examination of non-sterile products: Test for specified micro-organisms. Current version.
23. ISO 11737-1, Annex C. Sterilization of health care products – Microbiological methods – Part 1: Determination of a population of microorganisms on products, 2018.
24. Bernardini JN, Morales F, Schubert WW, Kazarians GA, Koukol RC. Employing a grinding technology to assess the microbial density for encapsulated organisms. NASA Technical Briefs 2012:13.
25. Bernardini JN, Koukol RC, Kazarians GA, Schubert WW, Morales F. Using a blender to assess the microbial density of encapsulated organisms. NASA Technical Briefs 2013:22–23.
26. Chee HY, Kim H, Lee MH. In vitro Antifungal Activity of Limonene against Trichophyton rubrum. Mycobiology 2009;37(3):243–243.
27. Yu LZX, Xiang WL, Huang M, Tang J, Lu Y, Zhao QH, Zhang Q, Rao Y, Liu L. Antifungal activity and mechanism of D-limonene against foodborne opportunistic pathogen Candida tropicalis. LWT-Food Science and Technology 2022;159:113144.
28. Sardessaia Y, Bhosleb S. Mini-review tolerance of bacteria to organic solvents. Research in Microbiology 2002;153:263–268.
29. Setlow P. Spore Resistance Properties. In: Driks A, Eichenberger P, editors. The Bacterial Spore: From Molecules to Systems. ASM Press eBooks, 2016; 201-215.
30. Schwarz R. Bioindicator – Practical Experiences. ECA Pre-Conference Workshop Bioindicators, 2016 May 10; Barcelona.
31. Schwarz R. Bioindikatoren in der Sterilisationskontrolle. Pharmazeutische Industrie 2020;82(1):112–123.
32. Geser J, Goverde M, Bohn C. Improved process understanding of the microbiological lethality during plastic extrusion as part of the Blow-Fill-Seal process for aseptic manufacturing. PDA Journal of Pharmaceutical Science and Technology 2025. In preparation.
33. EMA. Guideline on the sterilisation of the medicinal product, active substance, excipient and primary container. EMA/CHMP/CVMP/QWP/850374/2015, 2015.
Authors
Michael Karl Jahn¹, Marcel Goverde², Samira Giger², Natalie Reinhardt¹, Bernadette Bauer¹,
¹ Holopack Verpackungstechnik GmbH, Bahnhofstraße 18, D-74429 Sulzbach-Laufen, Germany
² MGP Consulting GmbH, Melchtalstrassse 21, CH-4102 Binningen, Switzerland
Corresponding Author: Michael Karl Jahn, Senior Manager Quality Control
Holopack Verpackungstechnik GmbH,
Bahnhofstraße 18,
D-74429 Sulzbach-Laufen, Germany
Email: Michael.Jahn@rommelag.com
Telephone: +49 7975 960 226
Comentarios