Opinion Article | Open Access | Published 16th July 2024
PHSS perspective on definitions and points to consider in efficacy claims of Sterilisation, Bio-decontamination, Disinfection, Cleaning, Decontamination when applied to Barrier technology
James Drinkwater | EJPPS | 292 (2024) | Click to download pdf
Introduction
The following are key points around definitions, regulatory expectations and connections between processes of Sterilization, Bio-decontamination, Disinfection, Cleaning and Decontamination applied to Barrier technology in GMP settings:
The perspective is from James Drinkwater, Honorary member and Ex-Chairman of the PHSS: Pharmaceutical & Healthcare Sciences Society and current co-lead of the PHSS Annex 1 implementation, Aseptic processing and Control Strategies Focus groups.
James is also the Head of GMP Compliance for Franz Ziel GmbH.
Acronyms and efficacy claim of vaporised hydrogen peroxide vapour
When discussing the process of bio-decontamination with vaporised hydrogen peroxide there are various acronyms used across the literature and in presentations including, VHP (Vaporised Hydrogen Peroxide – a considered trademark of Steris), VPHP (Vapour Phase Hydrogen Peroxide) and the more chemical symbol based vH₂0₂ (Hydrogen Peroxide sporicidal agent vaporised for application).
In this article the combined acronyms of VHP/ vH₂0₂ are applied to cover the variants of application that in common start with a hydrogen peroxide sporicidal agent solution that is flash evaporated into a condensable vapour (not a true gas) for application in bio-decontamination of surfaces within closed spaces i.e. Barrier technology: Isolators and Closed RABS, Material transfer devices and Cleanrooms. The efficacy of VHP/vH₂0₂ sporicidal reduction has proven efficacy to achieve 6log reduction of Geobacillus Stearothermophilus as biological indicator challenges (inoculated carrier in a Tyvek® primary pack).
Key points to consider of definitions and application on Barrier technology
Sterilisation is a penetrative process with method references in Pharmacopeia’s e.g. Moist Heat, Dry Heat, Gamma irradiation and ETO (Ethylene oxide, a true gas applied under vacuum). In variance to terminal product sterilisation the alternative of aseptic manufacturing applies sterilisation for sterilisation of both direct product contact parts (filling pumps, fluid paths and filling needles) and indirect product contacting surfaces e.g. container closure feed bowls, chutes and trackways. Without exception direct product contact parts must be sterilised by a pharmacopeial method. By default, in-direct product contact parts present a risk of sterile product contamination hence should also be subjected to a process that assures sterilisation of surfaces.
A key consideration for some time has been if such indirect product contact surfaces can be qualified as having sterile surfaces with an alternative process to a pharmacopeia penetrative method that achieves the required surface sterilisation condition. It is expected any alternative method must be applied following QRM principles. There has been open discussion and in some cases decisions to apply VHP/vH₂0₂ for ‘surface sterilisation’ with claims that the required 6log sporicidal efficacy is achieved and qualified via biological indicators, and such an alternative approach is considered to meet the requirements of QRM.
Importantly QRM and supportive risk assessments should never be considered to justify poor process or practice and in sterile product manufacturing any alternative method should meet the requirements and expectations of EU GMP Annex 1. Process understanding requires understanding of the difference between efficacy ‘claim’ and delivering a process that meets surface sterilisation on process critical surfaces (not just on biological indicators that are a proxy for the target surfaces).
Scientific knowledge and process understanding is fundamental to assurance of product sterility and patient safety as key requirements of QRM.
VHP/vH₂0₂ is not a ‘true’ sterilisation process as it has limited penetration capability hence appropriate as a surface treatment that only under certain conditions can achieve ‘surface sterilisation’ and certainly zero CFU recovery in environmental monitoring (EM). Both the limitation in VHP/vH₂0₂ penetration and EM recovery impact the efficacy claims made for surfaces in process environments on barrier technology and process equipment (non-product contact and indirect product contact surfaces).
Regulators have seen too much focus on biological indicators (BIs) efficacy claims without consideration of process limitations that may apply to the critical process surfaces in Grade A processing environments and on processing equipment. In efficacy qualification studies ‘Rogue’ BIs (BIs that have spore clumping and increased resistance to inactivation because of the lack of spore availability/exposure) underline the penetration limitation of VHP/vH₂0₂. In addition, soiled surfaces e.g. fatty acids from finger touch, if not properly cleaned before VHP/vH₂0₂ exposure may protect/shield biocontamination that could later be exposed in processing operations. Add to that the challenges of VHP/ vH₂0₂ distribution as a condensable vapour that is subjected to hydrogen bonding characteristics, hence poor at passive diffusion, the challenges to achieve surface sterilisation are not to be underestimated.
Without due regard of target surface exposure and inherent limitations of VHP/vH₂0₂ any claims of ‘surface sterility’ will not be valid. One of the main reasons the MHRA released the ‘Blog’ on ‘Fragility of VHP’ was not to characterise the bio-decontamination process as not suitable in application of aseptic manufacturing but to challenge the ‘fragility’ of scientific knowledge and process understanding for VHP/vH₂0₂ as a bio-decontamination process.
When the PHSS prepared the ‘Clarity of GMP Guidance note no.2; Assurance of Sterility of Indirect Product Contact Parts in Isolator Barrier Technology Aseptic Manufacturing’ and submitted it to the MHRA before publication, the PHSS were told this is exactly what was hoped would happen following the Blog, i.e. the industry started to think more about gaining and sharing scientific knowledge together with building process understanding of VHP/vH₂0₂ as a bio-decontamination process, so limitations and process variables are understood and risks managed by design, characterised for application and qualified to deliver a repeatable and robust bio-decontamination process that follows QRM principles.
Sterilisation vs Bio-decontamination with VHP/vH₂0₂
With good scientific knowledge and process understanding the limitations of VHP/vH₂0₂ can be managed and the strengths of VHP/vH₂0₂ can be fully realised.
The key strengths are considered as:
Bio-decontamination with the automated application of a sporicidal agent with broad-spectrum efficacy having oxidising potential and a free radical attack mechanism that inactivates, bacteria, fungi/moulds, spores and viruses (subject to DNA cleaving).
Cycle development via iterative studies can characterise inherent process variables to facilitate minimising variables to improve the process and provide a recipe for control parameters suitable for routine production use.
Efficacy can be qualified with biological indicator challenges and overkill applied to assure a robust and repeatable bio-decontamination process
Together with the efficacy the breakdown components of VHP/vH₂0₂ are the safe components of water and oxygen that are not persistent, hence can deliver a residue free bio-decontamination process, subject to starting agent elemental impurities and oxidising impact on resident residuals e.g. from cleaning agents.
For aeration and residual removal VHP/vH₂0₂ can be broken down by simple catalysts and dilution via venting to the outside environment where breakdown occurs to the safe components: oxygen and water. The same characteristics cannot be claimed for ‘true gases’ that readily diffuse and have lower OEL e.g. 0.1ppm.
As a condensable vapour VHP/vH₂0₂ that is poor at passive diffusion the vaporised agent can be managed and applied safely in closed spaces including within barrier technology and material transfer devices have a defined and qualified leak integrity with operators present in the surrounding environment. With an OEL of 1ppm any leakage is limited in terms of spread and exposure to operators.
The combined attributes of ‘Strengths’ of VHP/vH₂0₂ together with many peer-reviewed published articles relating to different microbiological species bio-decontamination and regulatory acceptance of a process capable of qualification by biological indicator efficacy challenges, means that VHP/vH₂0₂ still remains the most commonly applied bio-decontamination process for Barrier technologies, Material transfer devices and Cleanrooms.
VHP/vH₂0₂ is subjected to interconnected process variables and with the biphasic nature of the condensable vapour characteristics there is interaction between gas and liquid phases, adding another level of process variability and required process understanding when applied in aseptic manufacturing with use of barrier technologies. The term VHP gas generator misrepresents the biphasic nature of VHP/vH₂0₂ and has led to poor process integration treating ‘gas generation’ like a true gas and not considering the vapour will condense on route if the conditions are not managed.
Within the process environment there is a choice to make via ‘generator design’ and amount of VHP/vH₂0₂ flash evaporated whether the intent is to stay short of dew point or develop conditions past dew point where saturated vapour conditions are achieved. The so called ‘Dry or Wet’ VHP/vH₂0₂ bio-decontamination process. To address marketing claims of which was the better process, a scientific study was completed to examine the interactions and impact on efficacy of the process conditions of gas concentration, humidity/ temperature and condensation ᴿᵉᶠ ¹ Beatriz Unger-Bimczok ᵉᵗᵃˡ.
The outcome of the study discovered that it was better to generate bio-decontamination conditions past dew point where saturated vapour conditions led to the process of condensation: deposition and bonding of VHP/vH₂0₂ molecules on surfaces. However, it was found only 2-6 microns of deposition was required to achieve 6log sporicidal efficacy and any over injection or heavy condensation did not provide added efficacy. A deposition of 2-6 microns is invisible to the human eye, so in reality the optimum process is not ‘Wet or Dry’ as there is no visible wetting of critical process surfaces and as the process is past dew point it cannot also be considered as ‘Dry’. For such a process of surface deposition of VHP/vH₂0₂ it is also important to understand this is a dynamic process. In equilibrium saturated vapour conditions there are as many molecules of flash evaporated molecules arriving on the surface as leaving, so a dynamic exchange is required through the bio-decontamination phases to maintain efficacy.
For such processes applying saturated vapour micron deposition it should be recognised that where thermal gradients exist there may be different extents of visible deposition e.g., on a glass – glove port barrier from Grade A to the surrounding Cleanroom, e.g. Grade C for an Open System Isolator. Because of higher thermal gradients there may be visible ‘misting’ or so called ‘micro-condensation’ but on critical process surfaces if the cycle is optimised there should be no such visible deposition.
So, there are variants in the way the VHP/vH₂0₂ bio-decontamination process is integrated, the process variables are managed that deliver optimum efficacy and aeration times. One of the negative aspects of over-injection of flash evaporated VHP/vH₂0₂ is not just no added efficacy but also the increase in aeration times needed to remove gas residuals, both without benefit to the developed cycle. Again, process understanding for the variant selected and applied is a fundamental requirement to manage process variables that are related to the process in application.
USP (1229.11) Vapor Phase Sterilisation presents in the title the consideration Vapor applied sporicidal agents can achieve ‘Sterilisation’ without any reference to penetration limitations hence confusing the definition of sterilization and application. Reference is made to suitability as a ‘surface sterilisation’ method but without caveat this method is only appropriate as a surface treatment when applied as a vapor phase process because of the penetration limitations. In some material transfer devices vacuum can be applied to mitigate the penetration limitations but this is not a method that is suitable for barrier technology.
Extract <1229.11> Vapor Phase Sterilization
INTRODUCTION
Sterilisation can be accomplished using sporicidal agents suspended in air (i.e. vapor).
Sterilising agents that operate in this fashion include hydrogen peroxide ( H₂0₂), peracetic acid (CH3CO3CH), formaldehyde (CH20), and glutaraldehyde [CH2(CH2CHO)2] in aqueous solution. At room temperature these are liquids or solids that can be vaporized for introduction into a vessel or chamber. They differ from sterilizing gases and liquids in that there are multiple phases within the vessel during sterilization. Vapor sterilization systems are well suited for heat-sensitive materials and surface sterilization. Items exposed to the process should have their surfaces exposed to the greatest extent possible. Vapor sterilization processes require appropriate sterilant concentration, temperature, and relative humidity, all of which may be variable during the exposure period.
Comment: Text indicates an attention to surface exposure, and this defines ‘surface sterilisation’. There is no regulatory reference for ‘surface sterilisation’ with the term Bio-decontamination generally applied for gaseous – vapor phase-based processes used in barrier technology (for bio-decontamination of barrier, installed process equipment and load item surfaces) to differentiate from penetrative sterilization.
When citing other methods of ‘Vapor phase sterilisation’ no reference is made to the carcinogenic and corrosive nature of alternative methods to hydrogen peroxide vapor and the inherent challenge to control and monitor such processes (apart from corrosive impact on surfaces treated, monitoring instrumentations are highly impacted) – why even cite these inappropriate alternatives? There are alternatives in the way VHP/ vH₂0₂ vapour is delivered adding to another variable with no reference to these method variants in the introduction.
Process variables in Hydrogen peroxide vapor phase bio-decontamination cycles:
With a condensable vapour applied past dew point there are transitions when flash evaporated (generated) molecules of H₂0₂, in pico range 10-12 form a saturated vapour environment (‘molecules suspended in air’) and at saturation (when no more molecules can be held in the environment) surface deposition occurs leading towards formation of a condensate layer. Molecules merge and combine via hydrogen bonding and build to a layer on a surface e.g. micro-condensation (ideally below visible condensation) that can deliver 6log sporicidal efficacy.
Importantly the flash evaporated ultra-small VHP/vH₂0₂ molecules in the first instance (gas phase) have critical attributes as they can be distributed via methods that overcome the passive diffusion characteristics, can pass though filter media (HEPA filters and Tyvek®) and can form around point-contact supports of any loads presented for surface bio-decontamination. Once into the liquid phase where molecules merge and bond forming a micro-deposition layer there is targeted delivery of the sporicidal agent to critical surfaces and provided the dynamic exchange of gas molecules is maintained a high level of sporicidal efficacy (6log+) can be achieved.
However, this as this condensate layer formation is not ‘static’ (as that would be like spraying a disinfectant on a surface) the continuing dynamic exchange of molecules from the surface (in liquid phase) to the environment (in the gas phase) requires (optimally) the gas concentration in the process environment to be turbulent, facilitating molecule surface contact and exchange. The focus is on maintaining turbulent saturated vapour conditions and not purely on gas molecule distribution at a defined gas concentration that would be impacted by process variables and influenced by contact of complex process equipment surfaces and load patterns.
With temperature influences and distribution influences (particularly through injection of heated incoming vapour and method of achieving optimised distribution) conditions within an environment (chamber) can vary throughout the chamber. Such variations mean location to location efficacy can vary and process parameters and phase times for the worst-case location bio-decontamination (upon which overkill is applied) will be the subject of cycle development. Increasingly enzymatic indicators (EIs) support biological indicator (BIs) cycle efficacy qualification studies to characterise location to location efficacy and determine what are ‘worst case’ locations.
Attempting to remain in the gas phase (below dew point), so called dry process, with a condensable vapour as the sporicidal agent adds to further process complication (to balance variables) and process variability that can lead to challenging cycle development. Condensable vapour is influenced by temperature and humidity variations, and with incoming hot vapour and inherent variation in saturation, in-chamber gradients are created.
In-chamber variations require (optimising) minimising, so conditions are as homogenous as possible and a key function of achieving such an aim is effective distribution and temperature management. Such variations cannot be avoided but they can be optimised and that is part of cycle development characterisation – it is never all about just ‘killing BIs’.
Injecting vapour into unidirectional airflow in a circuit subjected to temperature variation (plenum and technical areas will vary from the Grade A processing zone) will not provide optimised conditions both in terms of mixing and molecule exchange with surfaces. High flow passing over a surface (particularly as air speeds up in entering air return(exhaust) points) can lead to worst case BI inactivation locations as residence time and molecule surface exchange are particularly challenging in high flow conditions.
The natural transitions between the gas and liquid phase of a vapour phase bio-decontamination process based on physical chemistry are understood and can be characterized, however inherent process variability has to be characterised and minimised to develop optimum and robust cycles. Cycle develop requires characterisation of a ‘Dose per phase’ (typically delivered as grams per minute) for a developed phase time as together with temperature and humidity they establish conditions for saturated vapour.
There are different vapour pressures between hydrogen peroxide and water and in transitions between forced flash evaporation at vapour generation through to the gas and liquid phases that apply in the target chamber result in surface condensate levels at a higher concentration (i.e. 60%) before the equilibrium state is developed i.e. equilibrium is when the surface condensate is the same concentration as the starting agent concentration (i.e. 35%).
Gas concentration: ppm ‘peaks’ either at establishment of saturated vapour conditions or at the start of the aeration process (gas residual removal) indicate the development of conditions where surface deposition occurs or commencement of surface re-evaporation. It is not expected gas concentration profiles will remain completely ‘flat’ at saturation and gas concentration levels (parts per million) will vary and not be the same in every cycle.
There are too many influences on gas concentration (parts per million; ppm) to achieve set/ controllable levels. What is important is that the profile develops to demonstrate and change from the ramp up of concentration (to saturated vapour) into plateau conditions where process efficacy is optimised, hence the gas concentration level of the plateau may vary cycle to cycle. Such variations mean monitoring gas concentration alone cannot provide clear acceptance criteria process efficacy conditions are established. In some cases, high gas concentrations (ppm) have not yielded repeatable sporicidal efficacy (because saturated vapour conditions are not reached, and distribution is highly variable) and low concentrations have delivered the required efficacy in optimised saturated vapour conditions – efficacy is never just a function of gas concentration e.g. the higher the gas concentration the greater the efficacy; this is not the case.
Without any gas concentration monitoring and characterisation of the gas concentration profile through the cycle phases one is ‘blind’ to process conditions as a ‘fingerprint’ profile and hence blind to deviation from this characterised profile – gas concentration monitoring is process and cGMP critical for VHP/vH₂0₂ bio-decontamination cycle qualification and assurance of cycle validity.
Vapor Phase Sterilization?
Continued: Extract: 〈1229.11〉 Vapor Phase Sterilization. ᴿᵉᶠ ²
INTRODUCTION
Because the agent is ordinarily supplied as an aqueous solution, moisture is introduced with the agent. The consequences of variation in these parameters may be localized differences in relative humidity, agent concentration, and condensation rates on the surfaces to be treated, resulting in variations in process lethality. The parameters to be established include sterilant amount (usually derived from injection quantities), relative humidity, and temperature.
There is no demonstrated correlation between gas phase conditions, surface conditions, and microbial kill. For this reason, online monitoring of vapor phase concentration is not widely utilized as a control parameter. Efforts to develop a standardized biological indicator for vapor systems have been hampered by the multiphasic nature of these sterilants. Selection of the appropriate biological indicator (BI) and resistance should be based on experimentation within the user's system. Only under well-defined, specific conditions (e.g., agent concentration, humidity level, temperature, substrate, and phase) can a reliable D-value be established (for a definition of D-value, see Sterilization of Compendial Articles 〈1229〉).
Comment: Process variability is recognised in USP (1229.11)
In the USA a sterilant is considered to be sporicidal in contrast to a disinfectant that has more limited efficacy and is applied to inactivate bacteria. A sterilant does not mean its use provides sterilization but the method of application (process) can provide 6log sporicidal efficacy. In Europe the Biocides Directive applies and all biocides used must be registered and qualified against a Type application. Failure to register an agent for a Type process could mean the agent is removed from the market (impacting supply of starting agent).
The fact that Geobacillus stearothermophilus biological indicators are used to qualify 6log sporicidal efficacy both in moist heat sterilization processes and vapor phase bio-decontamination processes does not mean the processes are equivalent and both can be claimed to provide sterilization. In vapour phase hydrogen peroxide processes, the sterilant is not multiphasic the process is biphasic (inherent gas and liquid phases). USP recognises the extent of process variables in vapour phase processes but provides little understanding on how even surface sterilization can be claimed for a vapour phase process. The focus on BI efficacy only does not lead to robust and repeatable cycles, as discussed, with characterizing (that requires process understanding) and minimizing process variables setting of (acceptable) CPP (Critical Process Parameters) ranges must be established. Such CPPs are considered to be in a ‘design space’ where variables operate and interact without compromise to efficacy.
The combination of characterising CPPs, understanding the impact of CPP variability, understanding the influences variables have on each other and BI efficacy challenges in different locations that demonstrate process lethality homogeneity, together with sub-lethal studies, are required to provide assurance that developed cycles are robust (with overkill margins) and repeatable for aseptic manufacturing operations.
Online gas phase (environmental) monitoring is essential in process monitoring. There is a correlation in physical chemistry with saturated vapour conditions, exhibited as a ‘plateau’ profile, and the cycle gas concentration profile (expressed in parts per million).
Although surface deposition or dynamic molecule exchange cannot be monitored these conditions are optimised for efficacy when saturated vapour conditions are reached (and monitored by development of gas concentration ‘plateaux). Once Dew point is reached (as physical chemistry this can be calculated) and saturated vapour conditions are achieved, continuation of vapour flash evaporation and injection leads to increasing the condensate layer. Over injection resulting in ‘heavy’ visible condensation is undesirable. ‘heavy’ condensation can also be a preferential influence on incoming gas molecules as there is a natural tendency to bond and develop equilibrium conditions, hence vapour molecule distribution can be affected.
Static surface deposition can be subject to catalytic breakdown (to components of water and oxygen) and as such reduce H₂0₂ concentration (efficacy). Even stainless steel is a mild catalyst, so H₂0₂ will not persist on surfaces.
Catalytic converters can be used to assist aeration (gas residual removal) with carbon impregnated media (with low pressure drop) having more than enough efficiency over precious metal catalysts beds that have a high pressure drop.
Without online monitoring of gas concentrations, the only other critical process parameters (CCPs) that can be monitored are starting conditions (temperatures and relative humidity), vapour injections rates that result in dosing amount per gassing phase and flow rates of the injected vapour delivery path. These parameters are not enough in process monitoring as primarily they monitor vapour generation and delivery but not the developing conditions in the target chamber/ volume. Without monitoring directly, the impact of dosing into the target environment (by online gas concentration monitoring) there is no detection of loss of delivery because of condensation on route. Other parameters that control vapour distribution within the chamber volume are also studied (with use of CIs; chemical indicators) and should be controlled and monitored.
Overkill is required to cover inherent and characterized process variables in vapour phase hydrogen peroxide bio-decontamination cycles. The ‘double cycle’ approach or % of additional cycle time does not work for vapour phase processes as this can lead to over saturation (heavy condensation) in the process environment. As heavy condensation adds nothing to surface efficacy overkill needs to be based on science and cover inherent process variables. Rationales should be prepared that define the approach (and calculation) in setting cycle overkill.
ETO Sterilisation vs Vapor Phase ‘Sterilization’
ETO applies a gas and vacuum process to deliver penetration of the sporicidal agent and hence meet requirements of penetrative sterilization. VHP sterilization chambers have been developed to apply vacuum to overcome the penetration limitations of VHP. Such vacuum chambers are suitable for medical device sterilisation, but such processes (including vacuum) cannot be applied to Barrier technology (Isolators and Closed RABS) and Cleanrooms.
Whether the complexities and amount of process variables associated with vapour phase hydrogen peroxide with the inherent biphasic nature (gas and liquid phases of a condensable vapour) can be qualified as a sterilization process that includes vacuum phases as an alternative to ETO is open to question. Research to characterise process variables and develop a qualification strategy is needed. As a sterilisation process together with proven efficacy, process monitoring will be required to provide the necessary assurance of sterility in applications e.g. medical device or Ready to Use sterile product containers (in Tyvek sterile barriers – Nested tubs).
Efficacy: 6log sporicidal efficacy and biological indication challenge studies in cycle qualification
Although 6log sporicidal efficacy is achieved on biological indicators subjected to VHP/ vH₂0₂ this is not a complete reflection of process efficacy. One of the main issues the MHRA observed related to VHP application was over claiming process efficacy (assumed sterility achieved with BI log reduction) without enough regard to the surfaces alongside the BI or gaps/ crevices/ mechanical spaces (including inside barrier gloves that are drawn back outside the Isolator) and generally at locations a BI could not challenge.
Surfaces could have bioburden, protective soiling and fatty acids from finger touch (that was not removed before load positioning in-place) hence with VHP/ vH₂0₂ and the penetration limitation all such surfaces could not be assured to achieve zero CFU under the protective layer even if the BI alongside indicated ‘surface sterility’ efficacy was reached. In short, the high attention to BI results without regard to the process conditions and VHP/ vH₂0₂ penetration limitation did not provide confidence in process understanding.
This lack of knowledge resulted in the MHRA Blog on ‘Fragility of VHP’ to get the industry thinking about the process and risks. The MHRA blog pointed out the risks and lack of process understanding around VHP bio-decontamination processes to encourage learning and knowledge development, so risked based claims and qualifications could be justified and applied in cGMP applications.
Rogue BIs are in the context of an atypical resistant BI that is inappropriate to challenge the efficacy of a surface bio-decontamination process where the limitations of VHP/ vH₂0₂ penetration apply. The presence of Rogue BIs, as a phenomenon that localised spore clumping as a result of BI manufacture (inoculation) demonstrate unexpected BI positives, needs to be considered and managed in cycle development.
Such ‘Rogues’ should be in low incidence; studies indicate in the range of 1% (independent studies to support current BI manufacturer claims) so in principle any cycle with more than 100 BIs applied may include a Rogue BI. Mass spore clumping or contaminated inoculum are not Rogue BIs, they are an out of specification BI lot. The point is that such bioburden loading (spore clumps) and unexpected BI positives support the risk concern about the penetration capability of VHP/ vH₂0₂ in the surface bio-decontamination process.
Bio-Decontamination is a combination of cleaning and high-level disinfection e.g. application of a sporicidal agent that can achieve 6log reduction. Qualified disinfectant agents are used and validated against laboratory efficacy challenges (coupon tests). The term ‘sterilant’ does not mean the agent can ‘sterilize’ but means the agent has sporicidal activity (and can typically also inactivate viruses) with a broader spectrum of biocidal efficacy than a general disinfectant.
The mode of action of hydrogen peroxide is via free radical attack and it possesses a high oxidizing potential delivering the broad-spectrum activity. Free radicals are powered to pair and in doing so can be extremely disruptive; penetrating cell walls/ spore layers, impacting cytoplasm and cleaving DNA (hence also virucidal).
Disinfectants are ‘rotated’ in a disinfection programme to increase the broadness in range of efficacy (combining agents with different modes of action). High concentration hydrogen peroxide sporicidal agents (typically 35% w/v and above) do not require rotation as there is already a broad spectrum of activity delivered.
Disinfectants have a limited efficacy and spectrum of activity and typically are applied to reduce bacteria and vegetative microorganisms to target levels e.g. 3 log reduction. Cleaning is required as a prerequisite step to assure the efficacy of the sporicidal agent on surfaces (hence bioburden and soiling removed to a level that will not impact the efficacy of the sporicidal agent).
For Isolators, Bio-decontamination is applied as an automated process to not only provide efficacy with a distributed sporicidal agent but to assure process variables are characterised/ managed and controlled within defined limits for set cycle phase times. Automated cycles reduce significantly the variability of agent application, distribution, residence and contact/ phase time that are challenged to apply/repeat in manual applications.
RABS Bio-decontamination, applied as a manual process must have the same intent of combined cleaning and high-level disinfection with a sporicidal agent that can achieve 6log reduction. In the case of Open RABS and Closed RABS (not subjected to VHP) then Bio-decontamination as a manually applied process requires appropriate risk mitigations to assure procedure controls meet the objectives.
Bio-decontamination of RABS has to achieve the Grade A conditions (Zero CFU recovery – no growth) inside the aseptic boundary of the Barrier technology that includes RABS gloves before aseptic manufacturing.
Annex 1 is clear in stating RABS gloves should be sterilized before installation onto the RABS Barrier system. Sterilisation (by a pharmacopeial method) can be onsite or by a supplier e.g. gamma irradiated, and here the protective packaging-wrapping plays a key role in maintaining sterility through to the point the packaging is opened and gloves installed on the barrier (at the filling line).
Annex 1 4.21 RABS: Gloves
For RABS, gloves used in the grade A area should be sterilised before installation and sterilised or effectively bio-decontaminated by a validated method prior to each manufacturing campaign. If exposed to the background environment during operation, disinfection using an approved methodology following each exposure should be completed. Gloves should be visually examined with each use, and integrity testing should be performed at periodic intervals.
Comment: At the point of RABS gloves installation (manual manipulation on to RABS Glove ports) typically in the first instance only of the exposed connection end of the glove (with most of the primary packing remaining in place) provides contamination limitation to a manageable level. Subsequent sporicidal disinfection of the limited surfaces put at risk of contamination from the Grade B surround and operator contact during glove installation can then re-establish full Grade A conditions in the full Barrier, including the gloves.
A subsequent Bio-decontamination (high level 6log sporicidal disinfection) would be also required prior to each manufacturing campaign if barrier doors would have been opened for set-up for a new batch of campaign (with gloves remaining on the Barrier).
In this case in-process cleaning may be limited to locations where product residues may be present with a cleaning agent that does not leave a residue e.g. alcohol based.
As we all know, open barrier door ‘intrusions’ during aseptic manufacturing should be avoided (by first intent) and if justified and qualified are expected as rare events. Aseptic processing intrusions are the highest risk intervention and any barrier door that is used in such an intrusion will require overhead L-UDAF (LAF) that covers the full swing of the barrier door that the gloves are attached to. Gloves may be protected (or drawn back from direct operator contact) to mitigate risks of recontamination.
As a final step following the intervention intrusion before the barrier door is closed a disinfection step should be applied: in this case only a disinfectant is applied to inactivate bioburden as cleaning is not expected as risks of soiling in this highly controlled process point is minimal.
H₂0₂ and variants in delivery/ distribution for process efficacy
There are variants in bio-decontamination processes that are automated or semi-automated but apply the same type of starting agent: H₂0₂ Hydrogen peroxide. Although there are alternative agents, the benefits of H₂0₂ having broad spectrum efficacy, simple break down to safe components of oxygen and water, and a free radical attack mechanism that provides a residue free process (properly controlled) still make this process the lead process of choice.
There is increasing knowledge how to manage residuals of the oxidizing agent when processing biological products and considerations of cycle end points (after aeration) may need to be below the OEL of 1ppm and in the parts per billion range because of bioproduct high sensitivities. However, process efficacy varies based on application method and concentration of applied H₂0₂ agent. The claims for one process, and published efficacy data cannot be taken as a given for another as process applications vary.
For an example VHP/vH₂0₂; In a process based on vapour generation (from a starting solution combining H₂0₂ and water) the flash evaporated vapour molecules are in the Pico range (10⁻¹²). In contrast, aerosol generation of droplets (dry fogging) is in the micron range. This understanding provides the challenge that VHP/vH₂0₂ molecules (in the gas phase) can pass through Tyvek and HEPA filter media, but aerosol droplets (at micron size) cannot.
In addition, in the liquid phase when molecules and droplets merge (bond) there can be no direct passage through filter media (Tyvek or HEPA). It may be possible to ‘wet’ the surface of Tyvek covering a BI and ‘wick through’ creating a high localized concentration yielding a rapid inactivation of the BI – but that is not the process with the intent to inactivate surface bioburden.
BIs are typically presented in the environment (not taped flat to surfaces) so gas phase molecules of vH₂0₂/VHP processes can pass through. There are in fact spores on the reverse of the inoculated BI carrier in the Tyvek so taping BIs flat to the surface is a poor practice as in any account it occludes sporicidal agent exposure to all spores. Also consider pull tabs of BI Tyvek are contaminated with spores (studies demonstrated that with Tyvek tabs cut off and separately incubated in TSB this resulted in positive growth. Tyvek pull tabs should not be taped down as ‘protective’ taping puts a risk of false positives in drop recovery in TSB tubes as a result of contaminating lab technician gloves/ fingers and subsequent handling cross contamination risks – good aseptic technique can only go so far to mitigate such risks.
Whether it is penetration limitations or agent delivery mechanism variants we just need to understand the process so limitations can be mitigated to acceptable levels of risk.
Disinfection. The terms disinfection and sanitisation can be considered interchangeable by process for GMP applications but are not considered interchangeable as terms of definition in language (different countries have different definitions and expectations of application of disinfection and sanitisation. Annex 1 has dropped the term sanitisation as in GMP disinfectants are qualified as agents with efficacy testing (including coupon testing) and applied in a disinfection procedure by an application method: spray, wipe, mop etc.
Procedures of application (SOPs) are qualified for manual application of a qualified agent. There are different types of disinfectant that require selection strategies based on GMP areas, application and criticality (extent of soiling and microbial profile that is the target). Disinfectant selection needs consideration to potential residues and requirements of sterility (that are required for higher grade cGMP areas).
Through material transfers enroute to Barrier technology a sporicidal disinfection step is required in a disinfection programme (process step location is commensurate with risk) and in other steps an IPA/ alcohol ‘disinfection’ step may apply that would remove/ inactivate some bioburden but without any sporicidal activity.
Cleaning; has a purpose of soiling or surface contaminant removal e.g. grease, lubricants, particulates; either by displacement (washing off) or collection on retentive media (wipes). Cleaning may be automated in-place (CIP) or through a manual procedure. In the case of wipes procedural control is required (critical areas first progressing to less clean areas with overlapping strokes). Some cleaning agents require a surfactant to remove bonded layers of soiling and in this case detergent agents are applied but such application will inevitably leave residues that may need a further cleaning step to remove residues with an agent that does not leave residuals e.g., alcohol based. Alcohol alone can ‘fix’ organic materials to surfaces.
Alcohol based cleaning agents are not sporicidal but when cleaning is required in combination with disinfection (one step application) for surfaces in controlled areas where recontamination/ soiling is limited (spores are not present) such agents can be used at a process step when a low (or no) residue process is required.
Cleaning is a pre-step to high level disinfection as part of the Bio-decontamination process.
Where cleaning is required for product residual removal where the product is toxic or biologically hazardous, cleaning validation is required. End points may need qualification based on health-based exposure limits (HBELs) where average daily exposure (ADEs) and permitted daily exposure (PDEs) apply.
Cleaning ahead of Bio-decontamination to establish ECs (Established Conditions) for manufacturing and cleaning ahead of decontamination (product residual removal) have very different objectives and qualification requirements. In cGMP applications the term ‘cleaning’ cannot be considered as a generic term and approach to cleaning and its purpose should be defined in the CCS.
Decontamination is considered as an end of batch process to remove product residuals. In contrast Bio-decontamination is specific for inactivation of bioburden/ microbial contamination and applied as a pre-step to establish conditions (ECs)³ for manufacturing products. Decontamination covers a wider range of contaminants, including product residuals that may be toxic, biologically hazardous or GMOs (e.g. viral vectors) in nature.
Where products are toxic chemical agents that can denature the product residual are required as the decontaminating agent. Where products are biological or vectors that can be inactivated by vH₂0₂/VHP a post-production cycle can be is applied.
(Reference 3; Established Conditions (ECs) ae referenced in ICHQ12 Life Cycle Strategies and in the context of Aseptic manufacturing they are the GMP Grade conditions that apply.)
Connection in a Contamination Control Strategy (CCS)
The combination of sterilization, bio-decontamination, disinfection, cleaning and decontamination for a given process needs to be defined in a CCS that considers their interactions and approaches taken as control measures.
Each step has specific qualification requirements that also need documenting in the CCS (strategy level and references).
Control strategies link through CMC and GMP aseptic manufacturing and such linkage should be understood. Product control strategies will combine many elements that cover different products and different levels of complexity in manufacturing. Although product control strategies developed for the CTD (Common Technical Document) and through CMC product development stages will consider requirements for contamination and cross contamination and requirements for containment measures, cGMP regulations require more in depth and documented strategies that are best defined in individual documents e.g. CCS, CCCS, ACS but with linkage (as the measures that apply to one impact another). The diagram below indicates the linkage:
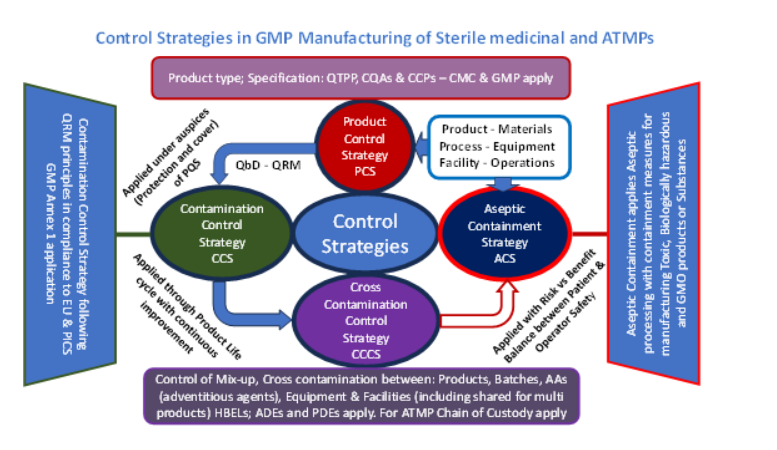
By default, sterilization, bio-decontamination, disinfection and supportive cleaning (for all steps) apply to the CCS prepared for an aseptic manufacturing process that follows QRM principles (ICHQ9(R1). Where QRM is applied to justify alternatives, any alternative should meet or be superior to the regulatory intent such that product quality, efficacy, patient safety and supply are not compromised e.g. maintained with acceptable levels of risk.
Decontamination may apply more directly in a cross-contamination control strategy (CCCS) where product residuals require risk mitigations for cross contamination between products, equipment, areas together with mitigating risks of product exposure to operators.
Where aseptic processing and containment are combined as process platforms that meet the CCS requirements for aseptic manufacturing of sterile medicinal and therapeutic products and operator protection and/or cross contamination control a complimentary aseptic containment strategy (ACS) may be developed. Such a strategy is based on OEB bands, HBELs and primary and secondary containment measures set by design.
Summary
Considering the various key points in this PHSS perspective related to sterilization, bio-decontamination, disinfection, cleaning, and decontamination, the importance of scientific knowledge and process understanding cannot be emphasized enough. Understanding individual processes with inherent variables is just the starting point as it follows how they interact (impact each other) and connect in control is a fundamental requirement to support the more holistic, risk based and proactive methodologies introduced in regulation revisions e.g. EU GMP & PICS Annex 1, ICHQ9(R1); QRM and ICHQ12; product life cycles.
The ’Black Box’ concept of purchasing a technology that delivers a ‘process’ without understanding the in-depth process and science behind the process is considered to be a position of the past. Such a situation relates to the vH₂0₂/VHP bio-decontamination process and how important it is to understand the variants, process variables and apply QRM principles in a given application. Gaining knowledge, building expertise will be important to successfully implement technologies, particularly if they are applied as ‘alternative’ methods. Control strategies are an important mechanism to document process and scientific understanding in applying contamination control measures and by narratives document the connections of control measures that together provide ‘collective effectiveness’.
Reference 1: The influence of humidity, Hydrogen peroxide concentration and condensation on the inactivation of Geobacillus stearothermophilus spores with Hydrogen peroxide vapor: Beatriz Unger-Bimczok et.al.
Reference 2. 〈1229.11〉 Vapor Phase Sterilization
Reference 3; ICHQ12 technical and regulatory considerations in product Life cycle strategies.
References
01. The influence of humidity, Hydrogen peroxide concentration and condensation on the inactivation of Geobacillus stearothermophilus spores with Hydrogen peroxide vapor: Beatriz Unger-Bimczok et.al.
02. 〈1229.11〉 Vapor Phase Sterilization
03. ICHQ12 technical and regulatory considerations in product Life cycle strategies.
Author Information
Corresponding Author: James Drinkwater, Head of GMP Compliance at F Ziel GmbH & ex-Chairman of PHSS - Head of Aseptic processing group
Franz Ziel GmbH

Comentarios