Peer Review Article | Open Access | Published 11th October 2024
Evaluation of a Real Time Airborne ‘Viables’ Particle Counter
T Eaton, K Capper, A Jones - AstraZeneca, Macclesfield, UK | EJPPS | 293 (2024) | https://doi.org/10.37521/29301 | Click to download pdf
Summary
A sampler that detects and counts ‘viable’ airborne particles in real-time was evaluated for potential use in cleanrooms used for aseptic manufacture. When tested within an environment deliberately enhanced with high numbers of microbe-carrying particles (MCPs), the sampler reported 4.8 times higher numbers of airborne ‘viables’ compared to the number of CFUs recovered by a traditional microbial air sampler and it is considered that this increased level may not be overly unrealistic. However, for similar testing within an environment enhanced with a high concentration of inanimate particles, but with extremely low levels of viable MCPs, the sampler reported 197 times higher numbers of airborne ‘viables’ compared to the number of recovered CFUs but it is considered that many of these were actually sourced from inanimate materials which intrinsically fluoresce and are therefore recorded as ‘viables’. If such a sampler is to be considered for cleanroom monitoring, comprehensive investigative work should be completed to determine the likely increase in the concentrations of reported ‘viables’ and the impact to the manufacturing operation, with the possibility of the introduction of scientifically justified limits, based upon the technology associated with real-time samplers, that, would need to be agreed with the relevant regulatory authority. For critical workstations, where the expectation is that no microbial contamination is recovered, investigative work to identify those inanimate materials that are reported to be ‘viables’ would need to be completed to provide a full understanding, so they can be eliminated or minimised. In these situations, real-time alert may be beneficial if the proportion of any implicated product could be immediately segregated, rather than waiting for the delayed reporting of any conventional CFUs, in which case, all of the manufactured product may have to be discarded.
Key words: Cleanroom; real-time microbial air sampler; ‘viables’, fluorescence; microbe-carrying particles (MCPs); airborne microbial contamination
1. INTRODUCTION
Real-time monitoring of airborne microbial concentrations within cleanrooms and associated controlled environments would provide considerable advantages over conventional sampling methods where there is a delay in obtaining results caused by the slow growth of microbes. This would be beneficial in aseptic pharmaceutical manufacture, where microbial excursions could almost immediately be detected and timely actions implemented with the additional possibility that the need to use traditional microbial air samplers and settle plates may also be negated. Furthermore, it is the expectation of the regulatory authorities that all available technology, that may enhance aseptic security, is appropriately investigated and utilised if appropriate¹. Previous investigations with this type of technology ²,³ reported that significantly more microbial contaminants (referred to in this paper as ‘viables’) were reported than recovered colony forming units (CFUs) associated with conventional microbial air samplers when sampling simultaneously in the same environment occupied by personnel. Additionally, particles generated from items such as sterilised cleanroom garments, sterilised 70% isopropyl alcohol in WFI (70% IPA), sterilised cleanroom wipes were also reported to be ‘viables’ where the traditional samplers recovered no CFUs. It was concluded that such airborne microbial concentrations were unrealistically high and this type of technology was not feasible for use within cleanrooms utilised for aseptic manufacture.
Further improvements of the technology have subsequently been progressed in an attempt to reduce the number of inanimate particles that are reported to be ‘viables’ and two different programmes of experimental work were completed to investigate this. The first programme utilised an environment enhanced with a high concentration of naturally occurring people borne airborne microbe-carrying particles (MCPs) and the second programme utilised an environment enhanced with predominantly inanimate airborne particles but with very few MCPs. The results from these two studies were then utilised to determine the extent of the increased recovery of ‘viables’ compared to the number of CFUs recovered by a traditional microbial air sampler, in order to provide information to determine the suitability of this type of technology for use with aseptic manufacturing cleanrooms. The experimental work was undertaken at AstraZeneca, Macclesfield, UK.
2. REAL-TIME ‘VIABLES’ PARTICLE COUNTERS
Available real-time monitoring systems for sampling airborne microbes are based upon measuring the quantity of light scattered by particles that fluoresce when induced by laser light at a specified wavelength. The fluorescence produced from certain microbial constituents can be measured by this method and the detection of a full range of micro-organisms, including viable and non-viable, aerobic and anaerobic, and those that are not cultured by normal methods, is potentially possible. The BioTrak® 9510-BD real-time viable particles counter was utilised for the investigations. This unit samples air at a rate of 28.3 L/min (1ft³/min) and particles that enter into the unit are firstly counted and sized in the same manner as a standard total particle counter. This total particle counter component has previously been compared with a standalone particle counter of known good performance and it was confirmed that comparable airborne concentrations were recovered ³ and so provides confidence that the BioTrak total particle counter function provides meaningful information. The air then enters a ‘particle concentrator’ which uses the principle of a ‘virtual impactor’ to concentrate the particles into 1.0 L/min of air, to reduce the air speed though the viability detector to obtain a better optical response. The remainder of the air is exhausted from the side of the concentrator, at a rate of 27.3 L/min, and passes through a HEPA filter before it is vented from the system. The viability detector measures the intrinsic fluorescence of microbial constituents, such as tryptophan, nictoinamide adenine dinucleotide (NADH) and riboflavin, that have been excited by a laser at a wavelength of 405nm. Any emitted fluorescence light is collimated, separated into two wavelength bands and collected by two photomultiplier tubes where the optical signals are converted to electrical signals. These are then processed using the ratio of the intensity of the two wave lengths and the light scattered to determine if a particle is ‘viable’ in nature. Those particles that are considered to be of ‘viable’ origin are counted and assigned to the following channel sizes (the standard total particles counter also reports at these same sizes);
≥0.5μm, ≥0.7μm, ≥1.0μm, ≥3.0μm, ≥5.0μm, ≥10.0μm
The unit utilised has two sensitivity settings, ‘normal’ and ‘high’, (note that the new instruments now have a single setting only), and the high sensitivity setting increases the likelihood that ‘viables’ are detected. After each minute, when 1 ft³ of air has been sampled, the total number of airborne particles at each channel size, and the number of ‘viables’ at the same channel sizes, are reported so that real-time information is continuously provided. Additionally, the sampler incorporates a gelatine-based filter (37mm diameter) and all of the air that has been optically analysed is passed over it, prior to discharge from the unit through the HEPA filter. The gelatine filter can be removed and transferred to microbiological growth medium and incubated for identification of any recovered colony forming units (CFUs).
Note; For clarification, the three types of (airborne) particles referred to in this paper are as follows;
Microbe-carrying particles (MCPs): The primary source of microbes in cleanroom air is personnel who disperse skin particles which carry one or more microbes, and are known as microbe-carrying particles (MCPs). MCPs that are capable of being cultured by conventional (active) microbial air sampling and are reported as CFUs and their airborne concentration determined from the volume of air sampled.
Total airborne particles: These particles are individually counted and sized by particle air samplers that use light scattering techniques. They are mainly inert particles but may include MCPs.
‘Viable’ particles: These particles are identified and counted by real-time microbial air samplers which analyse the fluorescence emitted by them to identify particles likely to be MCPs.
3. METHODS TO INVESTIGATE THE RECOVERY OF THE ‘VIABLES’ COUNTER COMPARED TO A TRADDITIONAL AIR SAMPLER
Real-time microbial air samplers may mistake particles that fluoresce for MCPs (false positives) and the failure to identify some MCPs (false negatives, which is also applicable to traditional methods) and this investigation was mainly concerned with the problem of false positives. Previous investigations were completed in well-ventilated cleanrooms and unidirectional airflow (UDAF) workstations, representative of the environments typically utilised for aseptic manufacture. However, the issue with this approach is that such environments have low levels of airborne contamination and the analysis of the resultant data is less meaningful than for that associated with an environment where larger concentrations of airborne contamination are present. With differences in responses to both MCPs and to inanimate particles reported by these real-time systems that utilise this technology, the investigations firstly examined the recovered number of ‘viables’ in environments where high concentrations of MCPs could be generated and then from a separate environment containing high concentrations of inanimate particles but with very few MCPs. Consequently, for Study 1, a high concentration of naturally occurring MCPs were generated from a technician exercising within a closed chamber and the concentrations measured simultaneously by the BioTrak unit and a conventional microbial air sampler. For Study 2, measurements were similarly taken in a non-unidirectional airflow (non-UDAF) isolator, following gas-in-place, where there were predominantly inanimate airborne particles generated from activities within the isolator, with very few viable MCPs present. The results from these two studies were then compared to determine the differences between the reported BioTrak ‘viables’ and the corresponding CFUs recovered by the conventional sampler to provide information regarding the suitability of the BioTrak unit for use during aseptic manufacture.
Study 1 – MCPS within a personnel chamber
This study was undertaken to focus upon the different responses to MCPs of the BioTrak unit and a conventional microbial air sampler. A redundant decontamination shower chamber, that has a front opening access door which can then be closed after entry and provides an environment of high numbers of airborne MCPs dispersed from a person in the chamber, was utilised and is shown in figure 1. Wearing street clothing, a technician performed light exercise in the chamber. The dispersed MCPs accurately reflect the actual type, size and shape of microbial flora within cleanrooms, unlike artificially generated droplets containing microbes that have been introduced into a test chamber, that may be damaged by the nebulisation introduction process and are likely to have a different fluorescence emission to naturally occurring MCPs found in cleanrooms. The conventional microbial air sampler used was an ImpactAir-140, slit-to-agar unit, with a rotating plate head that samples air at a rate of 28.3 L/min (1ft³/min) and uses 140 mm diameter tryptone soya agar (TSA) plates, with subsequent incubation for 5 days at 30-35⁰C. This sampler has been confirmed to be one of the most efficient available, with a biological collection efficiency of 254% when compared to the testing reference sampler 4. The resultant airborne contamination was simultaneously measured by the BioTrak unit and the ImpactAir microbial air sampler, located in adjacent positions within the chamber. Both instruments simultaneously sampled for three separate 36 minute periods to each provide three 1m³ samples (six in total), alternating the position of the samplers for the second test. When each of the 1m³ samples were concluded, the ImpactAir (TSA) plate and the BioTrak gelatine filter were changed and on completion of each of the three tests (1, 2, 3), the three gelatine filters were placed onto (sterile) 90mm TSA plates and incubated for 5 days at 30-35⁰C, alongside the three ImpactAir plates. The number of CFUs recorded per m³ by the ImpactAir sampler were then compared with the corresponding per m³ ‘viable’ counts reported by the BioTrak unit which also reported the corresponding numbers of total airborne particles for the same test period. The results are summarised in table 1 and as total airborne particles at the >0.5 μm and >5 μm sizes only are included in Annex 1 of the EU GGMP 1, only the concentrations relating to these two channel sizes are shown in table 1. Also included are the ratios for the CFUs recorded by the ImpactAir sampler with respect to the other reported results, and the ratios are also similarly shown for the BioTrak recorded ‘viables’.

Figure 1 Redundant decontamination shower chamber used to provide an environment of high numbers of MCPs
Study 2 – Inanimate particles within an isolator
This study was undertaken to focus upon any correlation between ‘viables’ recovered by the BioTrak unit and total airborne particles in an environment where the numbers of viable micro-organisms was reduced to a minimum but where the number of airborne inanimate particles was enhanced. This was achieved using a non-UDAF positive pressure, sterility testing half-suit isolator, that is shown in figure 2. It was loaded with low level bioburden product, microbial test media and equipment, closed and then any residual microbes were deactivated using a validated automated vapourised hydrogen peroxide (VHP) cycle. Non-UDAF is not as effective as UDAF for the control of airborne contamination and generated particles will stay airborne for prolonged periods of time and following the completion of the VHP decontamination cycle, a minimum number of viable airborne microbes would be expected to be present. A smaller ImpactAir-90 rotating plate sampler (used due to isolator space constraints), with the same high biological collection efficiency as the ImpactAir-140 mm unit ⁴ but utilising 90 mm TSA plates, operating at 28.3 L/min (1ft³/min), and the BioTrak unit (high sensitivity mode), were used at fixed adjacent locations. Three consecutive 36 minute periods were sampled to provide three separate 1m³ samples (6 samples in total) while a technician performed the typical activities undertaken for sterility testing. On completion of each of the three tests (3, 4, 5), the ImpactAir (TSA) plate and the BioTrak gelatine filter were changed. On completion of all three tests, the three gelatine filters were placed onto (sterile) 90mm TSA plates and were incubated for 5 days at 30-35⁰C, alongside the three ImpactAir plates. The number of CFUs recorded per m³ by the ImpactAir sampler were then compared with the corresponding per m³ ‘viables’ counts recorded by the BioTrak unit. Additionally, the ‘viables’ counts that were reported for each minute of the 36 minute test periods, as per ft³ samples, were matched with the specific technician activities that were undertaken in the same 1 minute sample period. Although it is not possible to definitively correlate activities with ‘viables’ counts due to the prolonged retention of airborne particles inside the isolator (associated with the isolator non-UDAF), it provides some information regarding the contamination generating events. The overall testing results are summarised in table 2 and as total airborne particles at the >0.5 μm and >5 μm sizes only are included in Annex 1 of the EU GGMP ⁵, only the concentrations at these two channel sizes are shown in this table. Also included are the ratios for the CFUs recorded by the ImpactAir sampler with respect to the other reported results, and the ratios are also similarly shown for the BioTrak recorded ‘viables’.
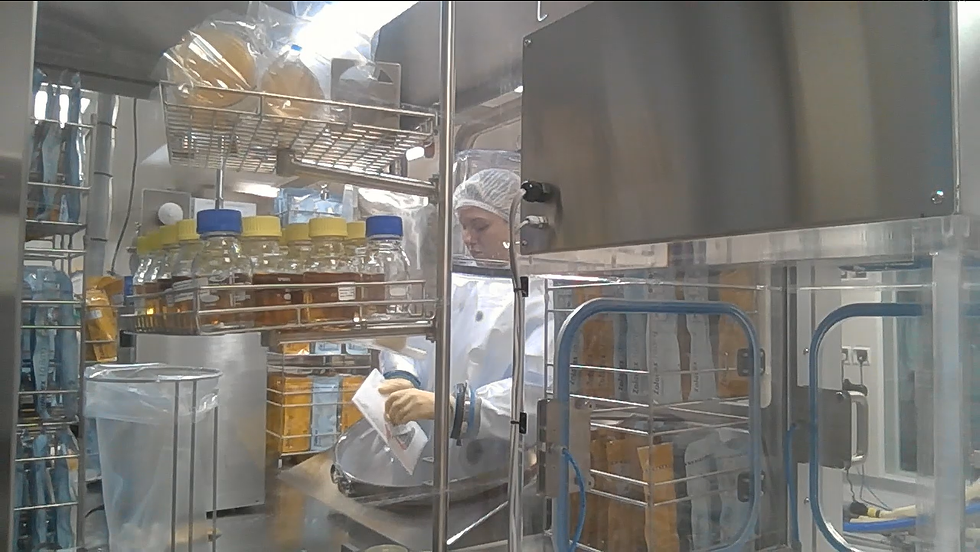
Figure 2 Non-UDAF gas-in-place sterility testing isolator
4. TEST RESULTS
Table 1 Study 1- summary of testing results from shower chamber
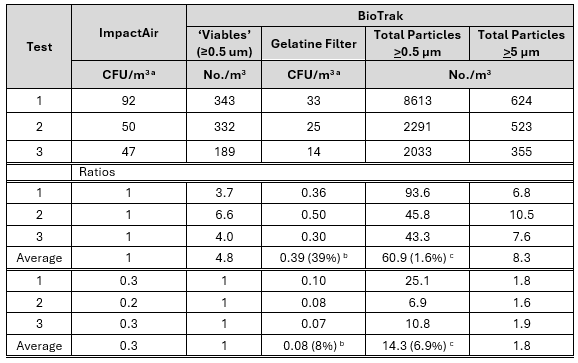
a. Bacteria and mould
b. Percentages in parenthesis relate to the number of CFUs recovered by the BioTrak gelatine filter as a proportion of the number of CFUs recovered by the ImpactAir sampler or ‘viables’ recorded by the BioTrak unit
c. Percentages in parenthesis relate to the number of CFUs recovered by the ImpactAir sampler or ‘viables’ recovered by the BioTrak unit as a proportion of the total airborne particles (>0.5 μm)
Table 2 Study 2 - summary of testing results from isolator

a. All bacteria
b. Percentages in parenthesis relate to the number of ‘viables’ recovered by the BioTrak unit as a proportion of the total airborne particles (>0.5 μm)
5. DISCUSSION OF RESULTS
For Study 1, the technician exercising in the shower chamber provided an environment with a high concentration of dispersed skin particles and a proportion of these contain microbes, known as MCPs, and are recovered onto the air sampler plates and, following incubation, those that are culturable, were reported as CFUs . On average, the BioTrak unit recorded 4.8 times more ‘viables’ than CFUs recovered by the conventional air sampler. This ratio is reflected by the proportion of the total airborne particles (particles >0.5 μm) that were recorded by the BioTrak unit to be ‘viables’ (6.9%) and the corresponding reduced proportion of CFUs (1.6%) recovered by the conventional sampler. This is in reasonable agreement with published information for people similarly exercising in a dispersal chamber, but wearing lint free cleanroom undergarments and using a less efficient conventional air sampler than used for Study 1, which determined that the recovered MCPs were less than 1% of the total airborne particles ⁵. However, it has been reported ⁶ that between 2. 2% and 54% of skin fragments dispersed into the air by people contain microbes. As microbes grow on the skin as microcolonies ⁷, the number on a skin cell or skin fragment is likely to vary from a single microbe to several hundred, although the average number of microbes on skin cells has been reported to be four ⁸. Shown in Figure 3 is an electro micrograph of the surface of skin with a microcolony containing about 30 bacteria. When airborne MCPs are sampled onto the solid TSA medium in the conventional sampler, each MCP gives rise to a single CFU irrespective of the number of viable microbes it may be carrying and so one CFU could be associated with many microbes (similarly, it should be noted that a single reported ‘viable’ may contain several cells). There is also the possibility that microbial cell debris, microbes that are not recovered by the conventional incubation conditions, and other inanimate intrinsically fluorescing clothing particles may also may be recorded by the BioTrak unit. Additionally, it is also important to note that as only 6.9% of the total (>0.5 μm) airborne particles were recorded by the BioTrak unit to be ‘viables’, it is likely that the BioTrak unit is capable of some level of differentiation between inanimate skin cells (ectodermal tissue) and skin cells with a microbial content and is unlikely to be recording the majority of these as ‘viables’. With consideration of all of these aspects, the 4.8 times increase in the number of recorded ‘viables’ compared to the corresponding number of CFUs, for this environment where there are high concentrations of human borne MCPs, may not be overly unrealistic.
It can also be seen that, on average, the gelatine filter recovered only 40% of the number of MCPs that were recovered by the ImpactAir sampler and only 10% of the corresponding number of reported ‘viables’. This reduced gelatine filter recovery is expected as it is only a simple component of the BioTrak unit that does not have the collection efficiency of the ImpactAir sampler, has a smaller filter area with the potential for multiple microbes to be deposited together and possible culturability issues following the subsequent transfer of the membrane to the microbiological growth medium. Therefore, it is considered that for cleanroom environments with low levels of airborne microbial contamination, only a small proportion of microbes that could subsequently be used for identification purposes may be recovered by the gelatine filter.

Figure 3 Microcolony of bacteria on surface of skin
Study 2 utilised an operational non-UDAF, positive pressure, half suit isolator that had been filled with typical sterility testing equipment and items and then decontaminated with VHP to provide an environment with very low numbers of MCPs but with high levels of inanimate particles. The results summarised in table 2 show that during operation, no MCPs were recovered by the ImpactAir sampler, but on average >197 ‘viables’ were reported by the BioTrak unit. On average, 3.8% of the total airborne particle concentration (particles >0.5μm) was recorded as ‘viable’ by the BioTrak unit, whereas no CFUs (0%) were recovered by the ImpactAir sampler for the same total airborne particle concentration. The 18 different operational activities that were completed during the testing periods are summarised in table 3 and shown in figure 4 are the specific technician activities that were undertaken in the same 1 minute sample period. The total airborne particle concentrations for each ft³ sample for each of the three tests, with the associated technician activities, is shown in table A1 in Attachment 1. It can be seen from figure 4 that when the isolator was in an ‘at rest’ condition with no activities being performed, and with the air supply and a positive pressure maintained, no ‘viables’ (and also no total airborne particles) were recorded. This provides information to confirm that the air supply, through the integral terminal (H14) HEPA filter, was contamination free and that there was no ingress of external contaminated air from the background isolator environment. However, all of the activities such as entering and exiting the half suit, positioning settle plates, moving waste bags and so on, generated particles that were recorded as ‘viables’ and so are likely to be directly related to these activities and not to the ingress of contaminated external air.
The components loaded into the isolator were known to have low bioburden concentrations and the VHP cycle has been demonstrated to be very effective in the deactivation of even the most resistant microbes. However, it may be possible that microbes de-activated during the VHP cycle that are subsequently dispersed into the isolator environment during the testing activities, and microbes that are not recovered by the conventional incubation conditions could be recorded as ‘viables’ by the BioTrak. However, with a recorded >197 times higher number of ‘viables’ reported by the BioTrak unit, it is likely that the majority of these types of ‘viables’ were actually sourced from inanimate testing materials and the isolator half suit fabric that intrinsically fluoresce and are therefore recorded as ‘viables’. These types of activities and materials utilised are synonymous with cleanroom manufacturing operations where similar responses to ‘viables’ would also be expected.
It should also be noted that two of the three gelatine filters recovered 3 CFUs between them. The reason for this is unclear and this highlights an issue with this feature, as the filter has to be removed and added to a TSA plate prior to incubation and this clearly adds additional risk to the aseptic security of the sampling method. For critical applications within workstations, it is unlikely, due to its size, that the BioTrak unit would be located within the workstation and typically, it would be located in the background cleanroom with appropriate tubing connecting it to the sampling point inside the workstation. Adequate cleanroom environmental protection within the cleanroom at this location would need to be provided to ensure that there is no filter contamination of the filter, or the TSA plate, during the filter removal and transfer process.
If the same portion of air that is sampled by a traditional microbial air sampler could be sampled by the BioTrak unit, and although not possible to prove, it may be reasonable to assume that the majority of microbes recovered by the traditional air sampler would be included in the larger number of ‘viables’ reported by the BioTrak unit. With this assumption, sampling with the BioTrak unit would report the worst case microbial concentrations compared to a traditional sampler and may therefore be a better option due to the real-time capabilities. However, for routine monitoring within pharmaceutical cleanrooms, the relative increases in concentrations of ‘viables’ could exceed the limits stated in the regulatory authority guides ¹,⁹. Although it would not be appropriate to apply these limits, as they relate to traditional sampling methods, they may need to be adhered to with likely to impact on overall cleanroom compliance levels and implications to the release of manufactured product. Alternatively, scientifically justified limits, based upon the technology associated with real-time samplers, that if possible correlate to the traditional CFU concentrations, would need to derived and agreed with the relevant regulatory authority. For extremely well controlled cleanroom
(EU Grade A ¹) workstations, such as those associated with isolators or restricted access barrier systems (RABS), the level of airborne microbial contamination is typically extremely low and conventional microbial air samplers very rarely recover any contamination. This presents more of a challenge as in this case, the regulatory authority expectation is that no such microbial contamination is recovered during sampling and the application of alternative real-time samplers derived limits, may not be acceptable. Low ongoing levels of total airborne particles (particles >0.5um) that intrinsically fluoresce may result in the reporting of ‘viables’ which would have implications to the release of manufactured product. Therefore, investigative work, to identify materials within such environments that fluoresce, would therefore need to be completed, and such materials would need to be eliminated or minimised. Nevertheless, accepting that there would likely be an increased number of ‘viables’ contamination, this technology may be of benefit for those applications where the proportion of the manufactured product implicated by the recording of any ‘viables’, in real-time, could be immediately segregated and discarded, rather than waiting for the delayed reporting of any CFUs associated with the conventional methods, where all of the manufactured product may then have to be discarded.
Table 3 Study 2 - technician activities performed in the isolator during sampling
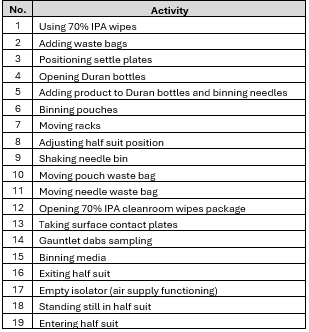

🟡 Technician activities associated with highest number of ‘viables’ (activities A to F, shown below)
Figure 4 BioTrak total ‘viables’ and the ImpactAir CFUs during isolator technician activities
Technician activities associated with highest number of ‘viables’ (refer to table 3 for full list of activities);
A – Placing settle plates, opening Durans bottles
B – Using 70% IPA wipes, opening Duran bottles, adding product to Duran bottles and binning needles
C – Opening Duran bottles, adding product to Duran bottles and binning needles
D – Adding product to Duran bottles and binning needles
E – Using 70% IPA wipes
F – Moving Racks
6. CONCLUSIONS AND RECOMMENDATIONS
The following has been concluded, based on the experimental work completed;
1. On average, within an environment where there were high concentrations of MCPs, the BioTrak unit reported 4.8 times higher numbers of airborne ‘viables’ compared to the number of CFUs recovered by a traditional microbial air sampler. In this environment, the BioTrak unit appears to be able to differentiate between human borne inanimate skin cells and those skin cells that have microbes attached to them. Consequently, a considerable proportion of the increased number of reported ‘viables’ could be associated with, for example, multiple microbes that form only a single CFU, microbial cell debris, de-activated microbes and microbes that are not recovered by the conventional incubation conditions, the reported increased number may not be overly unrealistic.
2. Similarly, within an environment where there were extremely low levels of viable MCPs, but a high concentration of other inanimate particles, on average, the BioTrak unit reported >197 times higher numbers of airborne ‘viables’ compared to the number of CFUs recovered by a traditional microbial air sampler. With this level of increased response, it is likely that the majority of these types of ‘viables’ were actually sourced from inanimate materials which intrinsically fluoresce and are therefore recorded as ‘viables’. This much greater (>41 times) response of the BioTrak unit to inanimate particles than to MCPs presents a greater issue for their use in cleanrooms and critical workstations used for aseptic manufacture that would need to be investigated, understood and addressed accordingly.
3. Assuming that sampling with the BioTrak unit would report the worst case microbial concentrations compared to a traditional sampler, it may be an appropriate option for cleanroom monitoring due to the real-time capability advantages. For cleanrooms where there are defined levels of allowable concentrations of airborne microbes, and in the absence of alternative scientifically justified limits, based upon the technology associated with real-time samplers, that have been agreed with the relevant regulatory authority, the limits that are specified by the regulatory authorities, may still need to be adhered to, and could be routinely exceeded and the use of the BioTrak unit may provide no advantages. For highly controlled critical (EU Grade A ¹) workstations, no airborne microbial contamination is the expectation and if the BioTrak unit was utilised, it is more likely inanimate particles would be reported as ‘viables’. Nevertheless, real-time alert may be beneficial if the proportion of any implicated product could be immediately segregated, rather than waiting for the delayed reporting of any conventional CFUs where all of the manufactured product may then have to be discarded. If this is not possible, utilisation of this technology is unlikely to be appropriate.
It is recommended that if the BioTrak unit is to be considered for cleanroom real-time monitoring, comprehensive investigative work should be completed to determine the likely increase in the concentrations of reported ‘viables’ and the impact to the manufacturing operation, as well as the consideration for implementation of alternative scientifically justified limits, based upon the technology associated with real-time samplers. For potential use within critical workstations, where the expectation is that no microbial contamination is recovered, investigative work to identify those materials that fluoresce, would therefore need to be completed and such materials are likely to be required to be minimised or eliminated from the workstation.
ACKNOWLEDGEMENTS
The authors are thankful to TSI incorporated for the loan of the BioTrak® 9510-bd real-time microbial air sampler.
CONFLICT OF INTEREST DECLARATION
The authors declare that they have no financial or non-financial conflicts of interest regarding this paper.
References
01. European Commission Guide to Good Manufacturing Practice, Annex 1:2022. European Commission, Enterprise Directorate-General, Brussels. 2022.
02. Eaton T, Davenport C and Whyte W. Airborne microbial monitoring in an operational cleanroom using an instantaneous detection system and high efficiency microbiological samplers. European Journal of Parenteral and Pharmaceutical Sciences 2012; 17 (2): 61-69.
03. Eaton T, Wardle C and Whyte W. Use of a Real-Time Microbial Air Sampler for Operational Cleanroom Monitoring. PDA Journal of Pharmaceutical Science and Technology 68(2): 172-184.
04. Eaton, T. Microbial Air Samplers for Meaningful Cleanroom Environmental Monitoring. European Journal of Parenteral and Pharmaceutical Sciences 26 (3), 2021.
05. Eaton T, Whyte W. Effective Re-usable Cleanroom Garments and Evaluation of Garment Life European Journal of Parenteral and Pharmaceutical Sciences 25 (4), 2021.
06. McIntosh C and Lidwell OM Towers AG, Marples R. The dimensions of skin fragments dispersed into the air during activity. Journal of Hygiene, Cambridge, 1978; 81: 471-479.
07. Somerville D A and Noble WC. Microcolony size of microbes on human skin. Journal of Medical Microbiology, 1972; 6: 323-328.
08. Lidwell OM, Noble WC and Dolphin GW. The use of radiation to estimate the numbers of micro-organisms in airborne particles. Journal of Hygiene, London. 1959; 57: 299-308.
09. Guidance for Industry Sterile Drug Products Produced by Aseptic Processing - Current Good Manufacturing Practice. US Food and Drug Administration, 2004.
APPENDIX 1
BioTrak recorded ‘viables’ concentrations and related isolator technician activities
Table A1 BioTrak recorded ‘viables’ and associated isolator technician activities
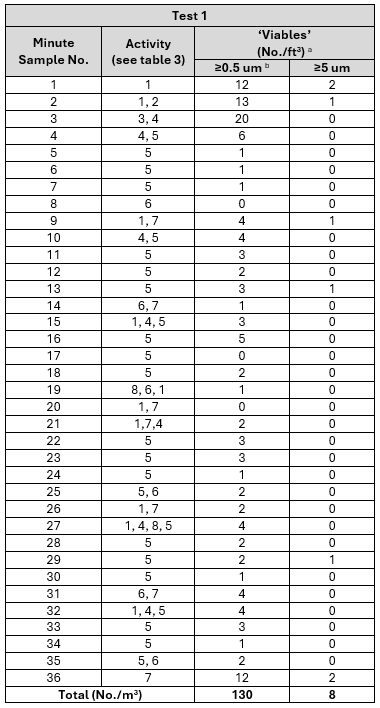
Table A1 BioTrak recorded ‘viables’ and associated isolator technician activities (continued)
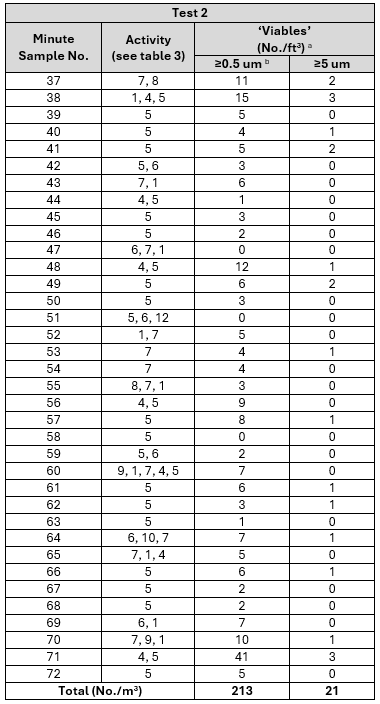
Table A1 BioTrak recorded ‘viables’ and associated isolator technician activities (continued)
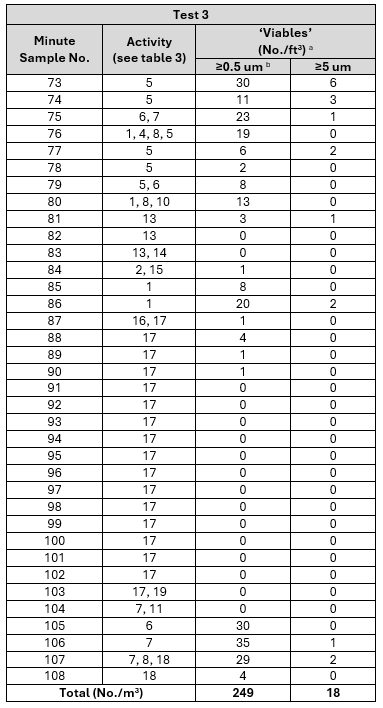
a. The BioTrak unit reports the counts for size channels ≥0.5μm, ≥0.7μm, ≥1.0μm, ≥3.0μm, ≥5.0μm and ≥10.0μm but as only the particles ≥0.5μm and ≥5μm are required by the EU GGMP Annex 1 to be measured, only these two sizes are included in the table
b. Particles > 0.5um represent the total ‘viables’.
Authors
T Eaton¹, K Capper¹, A Jones¹
¹ AstraZeneca, Macclesfield, UK
T Eaton, K Capper, A Jones AstraZeneca, Macclesfield, UK
Corresponding Author: Tim Eaton, Sterile Manufacturing Specialist
AstraZeneca,
UK Operations,
Silk Road Business Park,
Macclesfield
Cheshire. SK10 2NA
England
Email: tim.eaton@astrazeneca.com
Telephone: +44(0) 1625 514916
Comments